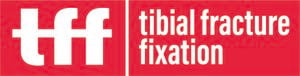
This module allows medical officers and surgeons who are not orthopedic specialists to become confident and competent in irrigation and debridement, powered and manual drilling, positioning and correctly inserting Schanz screws, and constructing the rod-to-rod modular frame as part of external fixation procedures for open tibial shaft fractures performed in regions without specialist coverage.
Overview[edit | edit source]
Global Impact[edit | edit source]
A systematic review of 204 countries estimated there were 455 million prevalent cases of acute or long-term symptoms of a fracture in 2019.[1]. The 2019 global prevalence of fractures of the patella, tibia or fibula, or ankle was 300 million cases resulting in an estimated 15.5 million years lived with disability (YLD). In the United Kingdom, two-thirds of open fractures of these bones were found to be fractures of the tibia or fibula.[2] Open tibial shaft fractures are the most common open long-bone fractures, and absolute fracture counts have been increasing substantially over the past 30 years.[1][3]
In low to middle income countries (LMICs), tibial fractures most often result from motor vehicle accidents, predominantly occur in young male adults (< 40 years, male to female ratio of 3:1), and often lead to reduced quality-of-life, loss of employment, and financial hardship for patients and their young families.[4][5][6] A 2018 Ugandan study found that only 12% of tibial fracture patients had recovered physically and economically at 24 months post-injury.
Access to high-quality orthopedic care in LMICs is limited by a lack of providers, resources, and training programs.[7] The global standard is a ratio of 1 orthopedic surgeon to 200,000 people.[8] In 2008, Uganda reported a ratio of 1 orthopedic surgeon for every 1.3 million people.[9] In 2020, Nigeria had an estimated population of over 206 million people.[10] According to the Nigerian Orthopaedic Association, Nigeria's ratio is 1 orthopedic surgeon to approximately 500,000 people.[8] An estimated 75% of Nigerian orthopedic surgeons are located in 4 major cities which leaves other urban areas and rural regions without coverage.[11]
The national shortages of orthopedic surgeons in LIMCs leaves patients vulnerable to traditional bone setters whose unsafe practices result in worse outcomes compared to no treatment and commonly lead to malunion, limb shortening, gangrene, limb loss, and death.[12][13][14][15][16] A 2007 study of Nigerian healthcare institutions found unacceptably high rates of amputation (57% to 77.8%) and mortality (11.1% to 26.7%) secondary to bone setter's gangrene.[12] A 2004 study on 82 Nigerian patients (median age of 27 years) found that the leading cause for limb amputation (32%) was gangrene resulting from treatment of extremity injuries by traditional bone setters.[13] Since medical officers do not receive adequate exposure to orthopedic surgery during their undergraduate medical education, they often refer fracture patients to traditional bone setters in regions without orthopedic specialist coverage.
In 2022, over 274 million people require humanitarian aid due to emergencies caused by conflict or natural disasters.[17] Nearly 45 million people in conflict zones lack basic access to healthcare.[18] Long bone fractures resulting from penetrating gunshot wounds account for over 25% of injuries sustained in conflict settings.[19] Conflict depletes an already overstretched surgical workforce, as doctors may become internally displaced or are forced to flee.[20] There is a lack of access to high quality surgical education in many conflict zones, as residency training programs either never existed or are closed down due to security risks, economic factors, and a lack of surgical mentors to oversee trainees. Surgeons operating in conflict-affected regions face targeted attacks, often with devastating consequences, which further reduces their numbers.[20][21] The security risks to healthcare workers in conflict zones pose a barrier to recruitment of local and international surgical staff.[20]
A 2017 review paper determined that 87% of injuries requiring treatment after earthquakes were orthopedic injuries, 65% of these injuries were fractures, 22% were open fractures, and the tibia/fibula was the most common fracture location (27%).[22] External fixator frames have been successfully used for orthopedic damage control for mass casualty events.[23][24] Over a 10 day period after the 2010 Haiti earthquake, orthopedists used external fixator frames to stabilize 72 lower extremity fractures (48 femoral fractures, 24 tibial/fibular fractures, and 1 humeral fracture) in a field-style operating room where limb alignment was evaluated via manual palpation, intraoperative imaging was not available, and soft tissue care was provided after bone stabilization.
In 2021, nearly 711 million people were in extreme poverty, which is defined as living on less than $1.90 per day.[25] This module's innovative, open-source, locally reproducible, low-cost, high fidelity, data-driven, gender-specific, labor-saving, eco-friendly, hygienic, and cruelty-free 3D printed bone simulators could empower local 3D printing entrepreneurs in low and middle income countries to build sustainable livelihoods.[26][27][28][29][30]
This self-assessed training module uses open source, locally reproducible, high fidelity 3D printed bone simulation models to educate and empower physicians who are not orthopedic specialists to perform external fixation procedures as part of the surgical management of open tibial shaft fractures to save limbs and lives. This module teaches essential irrigation and debridement, powered and manual drilling, and modular external fixation skills that are transferable to the performance of other limb-saving and life-saving surgeries that require hardware stabilization and fixation.[31] These skills can be used to prevent needless suffering, disability, and deaths for the estimated 133 million patients who sustain extremity and pelvic fractures globally every year.[1]
Open-Source 3D Printing Technologies for High Fidelity Orthopedic Surgery Simulation Training[edit | edit source]
Open-source 3D printing technology supports the local and automated reproduction of the highest fidelity bone simulation models at the lowest cost for medical officers and surgeons who are not orthopedic specialists in LMICs.
Open-source, open filament and user-friendly desktop 3D printers are currently in use at small to medium enterprises, Makerspaces (including but not limited to approximately 2,000 Fab Labs in over 149 countries), start-up incubators, universities, and hospitals worldwide.[26][27][28][29][30][32][33][34][35][36][37][38][39][40] Our 3D printed bone simulation models are designed to reduce simulator costs, simplify the simulator build, and minimize simulator assembly time for the learner.
This module provides an open source library of downloadable, 3D printed bone simulation models which accurately represent bone length and diameter, external contour and cross-sectional shape, bicortical anatomy, cortical hardness, cancellous bone porosity, and microstructure, and far cortex thickness for both genders at drilling sites for tibial shaft fractures.[41][42][43][44][45][46][47][48][49][50][51][52]
All of the module's 3D printed models can be locally reproduced on open source, open filament, user-friendly, fused deposition modelling, single extruder desktop 3D printers that print polylactic acid (PLA), a low-cost, biorenewable, and biodegradable plastic.[53][54][55][56][57] According to two filament manufacturers, 3D printed PLA at 100% infill has a Shore Hardness D value of 81D, 83D and 84D while independently measured Shore Hardness D values of 3D printed PLA samples range from 80D to 88D (n=12).[44][45][46][47] These Shore Hardness D values of 3D printed PLA are within the 3-sigma range for the Shore Hardness D measurements of 86.7D + 1.91D (ave. ± s.d., n=1815) for human cortical bone.[48]
To maximize the likelihood that these 3D printed models provide similar tactile feedback as human bone and do not foster the development of anti-skills, the bone simulation models are made from PLA, a plastic filament with a hardness level similar to cortical bone, which exhibits force and displacement ratios similar to artificial bone, and whose haptic feedback was rated as similar to bone during drilling by experienced maxillofacial surgeons and surgical residents.[44][45][46][47][48][53][58][59] These bone simulation models are digitally manufactured using customized settings to match age, gender, and bone site-specific cortical thickness values, and cancellous bone porosity values for the target patient population.[4][5][49][50][51][52]
We addressed the shortcomings of 3D printed bone simulation models which lack overlying soft tissue and skin simulation layers in teaching the performance of modular external fixation of an open tibial shaft transverse fracture by highlighting the steps that cannot be performed during simulation training but must be performed during the actual clinical procedure in the training objectives, knowledge objectives, and procedure steps of the skills training module page and the checklist of the training logbook module page. The user's learnings on high fidelity 3D printed bone simulation models will translate into the clinical performance of Modular External Fixation for an Open Tibial Shaft Transverse Fracture which is described in 48 learning objectives on the skills training module page.
Pre-Learning Clinical Confidence Assessment[edit | edit source]
Before the learner starts this module:
- Go to this link.
- Download, print out, and complete the pre-learning clinical confidence assessment for this training module.
- Photograph the completed assessment on your cellphone as a backup and file the assessment in your training records.
Phase 1: Knowledge Review[edit | edit source]
It is highly recommended that the learner be familiar with this content before proceeding to the skill pages.
- Knowledge Review
- Pre-Operative Management
- Modular External Fixation
- Post-Operative Management
- Follow-Up Care
Phase 2: Simulator Build[edit | edit source]
3D Printed Adult Male Tibial Bone Models[edit | edit source]
The advantage of using 3D Printed Adult Male Tibial Bone Models is that these models are representative of the patient population that the surgical practitioner is most likely to encounter in real clinical scenarios because tibial fractures predominantly occur in young male adults (male to female ratio of 3:1).[4][5] The drawback is the relatively higher cost of the 3D Printed Adult Male Tibial Bone Models due to the greater amount of 3D printed filament required for the thicker far cortex of male adults.[52]
Instructions for 3D Printing Organizations[edit | edit source]
Please pay attention to and follow all the instructions closely to ensure the bone models are printed properly and display the required visual, tactile, and acoustic fidelity for orthopedic surgical simulation training.
- Confirm 3D Printing and Delivery Capabilities
- Download 3D Print Files
- Prepare 3D Print Files
- Check Print Settings
- 3D Print Sample Models
- Calculate Price Quote
- Inspect Models Before Delivery
Simulator Assembly[edit | edit source]
Surgical Hardware[edit | edit source]
Any locally available 4.5 or 5.0 mm diameter self-drilling Schanz Screws and compatible modular external fixator hardware, instruments, and surgical drill can be used for this skills training module.
Phase 3: Skills Practice[edit | edit source]
Phase 4: Self-Assessment[edit | edit source]
The Tibial Shaft Transverse Fracture Simulator is designed to include mechanisms for targeted feedback which enables the user to: ensure they are practicing the appropriate skills; modify their performance to improve competence; and determine when they have practiced to a sufficient level of mastery to perform the procedure in a patient.
The transparent cellophane that is wrapped around the 3D printed bone models and simulates the overlying periosteum will permit the learner to visually inspect and confirm post-fixation fracture alignment, the Schanz screws did not perforate the far cortex of the Tibial Shaft Transverse Fracture Simulator, and the Schanz screws are properly positioned medial to the tibial crest for the self-assessment framework.
Unlike conventional artificial bone fracture models, the base of the 3D printed models of the Tibial Shaft Transverse Fracture Simulator allows the proximal and distal fracture fragments to be positioned on a flat surface to permit easy, convenient, and precise measurement of the drill trajectory angles of the Schanz screws using a low-cost (20¢ USD) protractor for the self-assessment framework.[60]
The Training Logbook and Self-Assessment Framework include:
- Step-by-step procedural checklist to confirm following of the proper modular external fixation technique for open tibial shaft fractures
- Visual inspection and taking cellphone photos ("digital X-rays") to verify post-fixation fracture alignment, no pin penetration of the far cortex, proper pin positioning in each fracture fragment, and drill trajectory angles are within the acceptable ranges for the safe zones of the tibia
Based on our user testing, we opted not to use Far Cortex Breakthrough Detection or Plunge Depth Measurement for the Tibial Shaft Transverse Fracture Simulator. The rationale for these design choices is becauseː
- the likelihood of perforating the far cortex and plunging is very low with the manual advancement of the Schanz screw into the far cortex
- the transparent cellophane allows the learner to visually inspect and confirm that the self-drilling Schanz screws did not perforate the far cortex
- plunge detection has not been shown to confer long-term learning benefits in reducing plunge
- we want to avoid fostering learner dependence on augmented feedback ("anti-skills") since the learner will not have augmented feedback during the real procedure, and
- this increases simulator fidelity, reduces simulator costs, simplifies the simulator build, and minimizes simulator assembly time for the learner.[61][62]
Training Module Certificate of Completion[edit | edit source]
Once the self-assessment framework has been completed:
- Go to this link.
- Click on "Get your certificate" button under the "Menu" section in the upper right corner of the module page.
- Type in your name, download and print out a certificate of completion for this training module.
- Photograph your certificate on your cellphone as a backup and file the printed certificate in your training records.
Post-Learning Clinical Confidence Assessment[edit | edit source]
After the learner completes this module:
- Go to this link.
- Download, print out, and complete the post-learning clinical confidence assessment for this training module.
- Photograph the completed assessment on your cellphone as a backup and file the assessment in your training records.
If the learner does not feel confident in performing this procedure in real clinical scenarios, the learner can repeat this module and practice the skills training as many times as they feel necessary to gain the confidence to perform this procedure on patients.
Supplemental Learning Topics[edit | edit source]
(Optional) After completion of the module, the learner may wish to learn more about 3D printing technology:
Additional Module Information[edit | edit source]
(Optional) After completion of the module, the learner may wish to learn more about this module:
Follow-on[edit | edit source]
(Optional) After completion and practice to competency, the learner may wish to continue study with these courses:
- Uniplanar External Fixation
- Humeral Fracture Fixation
- Pediatric Distal Forearm Fractures
- Bicortical Drilling
Acknowledgements[edit | edit source]
This work is funded by a grant from the Intuitive Foundation. Any research, findings, conclusions, or recommendations expressed in this work are those of the author(s), and not of the Intuitive Foundation.
References[edit | edit source]
- ↑ 1.0 1.1 1.2 Global, regional, and national burden of bone fractures in 204 countries and territories, 1990–2019: a systematic analysis from the Global Burden of Disease Study 2019. GBD 2019 Fracture Collaborators. Published:August 20, 2021. DOI:https://doi.org/10.1016/S2666-7568(21)00172-0
- ↑ Charles M. Court-Brown, Ben Caesar, Epidemiology of adult fractures: A review, Injury, Volume 37, Issue 8, 2006, Pages 691-697, ISSN 0020-1383, https://doi.org/10.1016/j.injury.2006.04.130.
- ↑ Elniel AR, Giannoudis PV. Open fractures of the lower extremity: Current management and clinical outcomes. EFORT Open Rev. 2018 May 21;3(5):316-325. doi: 10.1302/2058-5241.3.170072. PMID: 29951271; PMCID: PMC5994617.
- ↑ 4.0 4.1 4.2 Omoke NI, Ekumankama FO. Incidence and Pattern of Extremity Fractures seen in Accident and Emergency Department of a Nigerian Teaching Hospital. Niger J Surg. 2020 Jan-Jun;26(1):28-34. doi: 10.4103/njs.NJS_42_19. Epub 2020 Feb 10. PMID: 32165833; PMCID: PMC7041343.
- ↑ 5.0 5.1 5.2 Babalola OM, Salawu ON, Ahmed B A, Ibraheem G H, Olawepo A, Agaja S B. Epidemiology of traumatic fractures in a tertiary health center in Nigeria. J Orthop Traumatol Rehabil [serial online] 2018 [cited 2021 Jul 1];10:87-9. Available from: https://www.jotr.in/text.asp?2018/10/2/87/245994.
- ↑ O'Hara NN, Mugarura R, Potter J, Stephens T, Rehavi MM, Francois P, Blachut PA, O'Brien PJ, Mezei A, Beyeza T, Slobogean GP. The Socioeconomic Implications of Isolated Tibial and Femoral Fractures from Road Traffic Injuries in Uganda, The Journal of Bone and Joint Surgery: April 4, 2018 - Volume 100 - Issue 7 - p e43. doi: 10.2106/JBJS.17.00439.
- ↑ Conway DJ, Coughlin R, Caldwell A, Shearer D. The Institute for Global Orthopedics and Traumatology: A Model for Academic Collaboration in Orthopedic Surgery. Front Public Health. 2017 Jun 30;5:146. doi: 10.3389/fpubh.2017.00146. PMID: 28713803; PMCID: PMC5491941.
- ↑ 8.0 8.1 Nigeria has 350 orthopaedic surgeons for 170 million citizens. (2016, November 26) Agency Report. Premium Times. Retrieved November 11, 2020, from https://www.premiumtimesng.com/news/more-news/216415-nigeria-350-orthopaedic-surgeons-170-million-citizens.html.
- ↑ Naddumba EK. Musculoskeletal trauma services in Uganda. Clin Orthop Relat Res. 2008 Oct;466(10):2317-22. doi: 10.1007/s11999-008-0369-2. Epub 2008 Jul 16. PMID: 18629599; PMCID: PMC2584282.
- ↑ Population, total - Nigeria [Internet]. Data. The World Bank; 2021 [cited 2021 Dec 10]. Available from: https://data.worldbank.org/indicator/SP.POP.TOTL?locations=NG.
- ↑ National Hospital records high patronage on knee, hip replacement surgeries. (2020, January 26) The Sun Nigeria. Retrieved November 11, 2020 from https://www.sunnewsonline.com/national-hospital-records-high-patronage-on-knee-hip-replacement-surgeries/.
- ↑ 12.0 12.1 Nwadiaro HC. Bone setter's gangrene. Nigerian Journal of Medicine Vol. 16 (1) 2007: pp. 8-10. DOI: https://doi.org/10.4314/njm.v16i1.37273.
- ↑ 13.0 13.1 Umaru RH, Gali BM, Ali N; (2004) Role of inappropriate traditional splintage in limb amputation in Maiduguri, Nigeria. Annals of African Medicine, 3(3):138-140. URL: http://www.bioline.org.br/request?am04034.
- ↑ Dada A, Giwa SO, Yinusa W, Ugbeye M, Gbadegesin S. Complications of treatment of musculoskeletal injuries by bone setters. West Afr J Med. 2009; 28(1):43–7.
- ↑ Mohamed Imad A, Hag EL, Osman Bakri M, Hag EL. Complications in fractures treated by traditional bonesetters in Khartoum, Sudan. Khartoum Med J. 2010;3(1):401–5.
- ↑ Onyemaechi NOC, Onwuasoigwe O, Nwankwo OE, Schuh A, Popoola SO. Complications of musculoskeletal injuries treated by traditional bonesetter in a developing country. Indian J Appl Res. 2014;4(3):313–6.
- ↑ https://gho.unocha.org/
- ↑ Creating Hope in Conflict: A Humanitarian Grand Challenge - Request for Proposals February 19, 2018.
- ↑ Bauhahn G, Veen H, Hoencamp R, Olim N, Tan ECTH. Malunion of Long-Bone Fractures in a Conflict Zone in the Democratic Republic of Congo. World J Surg. 2017 Sep;41(9):2200-2206. doi: 10.1007/s00268-017-4008-5. PMID: 28488041.
- ↑ 20.0 20.1 20.2 Adapted from: https://humanitariangrandchallenge.org/wp-content/uploads/2021/01/Analysis-of-Barriers-Affecting-Innovation-in-Humanitarian-Contexts.pdf
- ↑ International Committee of the Red Cross. Why we can't save her life | On The Frontline. [Internet]. San Bruno (CA): Youtube; 2018 May 3 [cited 2021 Aug 27]. Available from: https://www.youtube.com/watch?v=wm0TYebjyHQ.
- ↑ MacKenzie JS, Banskota B, Sirisreetreerux N, Shafiq B, Hasenboehler EA. A review of the epidemiology and treatment of orthopaedic injuries after earthquakes in developing countries. World J Emerg Surg. 2017 Feb 10;12:9. doi: 10.1186/s13017-017-0115-8. PMID: 28203271; PMCID: PMC5301447.
- ↑ Lebel E, Blumberg N, Gill A, Merin O, Gelfond R, Bar-On E. External fixator frames as interim damage control for limb injuries: experience in the 2010 Haiti earthquake. J Trauma. 2011 Dec;71(6):E128-31. doi: 10.1097/TA.0b013e3182147654. PMID: 21502876.
- ↑ Bar-On E, Lebel E, Kreiss Y, Merin O, Benedict S, Gill A, Lee E, Pirotsky A, Shirov T, Blumberg N. Orthopaedic management in a mega mass casualty situation. The Israel Defence Forces Field Hospital in Haiti following the January 2010 earthquake. Injury. 2011 Oct;42(10):1053-9. doi: 10.1016/j.injury.2011.03.054. Epub 2011 Apr 19. PMID: 21507401.
- ↑ Mahler DG, Yonzan N, Lakner C, Aguilar RAC, Wu H. Updated Estimates of the Impact of Covid-19 on Global Poverty: turning the corner on the pandemic in 2021? [Internet]. World Bank Blogs. 2021 [cited 2021 Dec 10]. Available from: https://blogs.worldbank.org/opendata/updated-estimates-impact-covid-19-global-poverty-turning-corner-pandemic-2021.
- ↑ 26.0 26.1 https://world.prusa3d.com/
- ↑ 27.0 27.1 https://3dafrica.org/
- ↑ 28.0 28.1 https://impacthub.net/about-us-regions-locations/
- ↑ 29.0 29.1 AIGE Limited. 3D printers. [Internet]. 3D Printers | AIGE Limited. [cited 2021 July 29]. Available from: https://www.aige.info/3d-printers.
- ↑ 30.0 30.1 MoTIV. Motiv tribe [Internet]. MoTIV. 2021 [cited 2021 July 29]. Available from: https://motiv.africa/.
- ↑ Debas, H. T., P. Donkor, A. Gawande, D. T. Jamison, M. E. Kruk, and C. N. Mock, editors. 2015. Essential Surgery. Disease Control Priorities, third edition, volume 1. Washington, DC: World Bank. doi:10.1596/978-1-4648 -0346-8. License: Creative Commons Attribution CC BY 3.0 IGO.
- ↑ https://www.creality3dofficial.com/products/official-creality-ender-3-3d-printer?gclid=EAIaIQobChMIn76Xn4_A9wIVD4FaBR0klwSJEAAYAyAAEgIzRvD_BwE
- ↑ Prusa J. Open-Source 3D printers from Josef Prusa [Internet]. Prusa3D. 2019 [cited 2021 July 29]. Available from: https://www.prusa3d.com/.
- ↑ https://lulzbot.com/store/sidekick747?ref=null
- ↑ Ultimaker. Ultimaker 3D printers: Reliable and easy to use [Internet]. ultimaker.com. [cited 2021 July 29]. Available from: https://ultimaker.com/3d-printers.
- ↑ https://www.fablabs.io/labs/map
- ↑ https://live.fablabs.io/
- ↑ https://wiki.hackerspaces.org/List_of_Hacker_Spaces
- ↑ https://makerspaces.make.co/
- ↑ Niatech. CoRSU Starts Field Testing 3D Printed Orthopaedic Devices For Children With Disabilities [Internet]. Canada: Niatech; June 13, 2016 [cited 2020 Nov 11]. Available from: https://niatech.org/corsu-starts-field-testing-3d-printed-orthopaedic-devices-for-children-with-disabilities/.
- ↑ Ugochukwu EG, Ugbem LP, Ijomone OM, Ebi OT. Estimation of Maximum Tibia Length from its Measured Anthropometric Parameters in a Nigerian Population. J Forensic Sci Med [serial online] 2016 [cited 2021 Jun 27];2:222-8. Available from: https://www.jfsmonline.com/text.asp?2016/2/4/222/197928.
- ↑ U.S. Department of Health and Human Services — National Institutes of Health. Human tibia and fibula. [Internet]. Bethesda, (MD): NIH 3D Print Exchange; 2014 May 29 [cited 2021 Aug 17]. Available from: https://3dprint.nih.gov/discover/3DPX-000169.
- ↑ Gosman JH, Hubbell ZR, Shaw CN, Ryan TM. Development of cortical bone geometry in the human femoral and tibial diaphysis. Anat Rec (Hoboken). 2013 May;296(5):774-87. doi: 10.1002/ar.22688. Epub 2013 Mar 27. PMID: 23533061.
- ↑ 44.0 44.1 44.2 www.prusa3d.com/file/370474/technical-data-sheet.pdf
- ↑ 45.0 45.1 45.2 Ultimaker. Ultimaker PLA Technical Data Sheet [Internet]. Ultimaker Support. [cited 2021 July 29]. Available from: https://support.ultimaker.com/hc/en-us/articles/360011962720-UltimakerPLA-TDS.
- ↑ 46.0 46.1 46.2 https://support.ultimaker.com/hc/en-us/articles/360011962720-Ultimaker-PLA-TDS
- ↑ 47.0 47.1 47.2 Vian, Wei Dai and Denton, Nancy L., "Hardness Comparison of Polymer Specimens Produced with Different Processes" (2018). ASEE IL-IN Section Conference. 3. https://docs.lib.purdue.edu/aseeil-insectionconference/2018/tech/3.
- ↑ 48.0 48.1 48.2 Society For Biomaterials 30th Annual Meeting Transactions, page 332. Femoral Cortical Wall Thickness And Hardness Evaluation. K. Calvert, L.A. Kirkpatrick, D.M. Blakemore, T.S. Johnson. Zimmer, Inc., Warsaw, IN.
- ↑ 49.0 49.1 Meyers, M. A.; Chen, P.-Y. (2014). Biological Materials Science. Cambridge: Cambridge University Press. ISBN 978-1-107-01045-1.
- ↑ 50.0 50.1 Forrest AM, Johnson AE, inventors; Pacific Research Laboratories, Inc., assignee. Artificial bones and methods of making same. United States patent 8,210,852 B2. Date issued 2012 Jul 3.
- ↑ 51.0 51.1 National Institutes of Health Osteoporosis and Related Bone Diseases National Resource Center. What is Bone? [Internet]. Bethesda (MD): The National Institutes of Health (NIH); 2018. [Cited 2021 Aug 17]. Available from: https://www.bones.nih.gov/health-info/bone/bone-health/what-is-bone.
- ↑ 52.0 52.1 52.2 Maeda K, Mochizuki T, Kobayashi K, Tanifuji O, Someya K, Hokari S, Katsumi R, Morise Y, Koga H, Sakamoto M, Koga Y, Kawashima H. Cortical thickness of the tibial diaphysis reveals age- and sex-related characteristics between non-obese healthy young and elderly subjects depending on the tibial regions. J Exp Orthop. 2020 Oct 6;7(1):78. doi: 10.1186/s40634-020-00297-9. PMID: 33025285; PMCID: PMC7538524.
- ↑ 53.0 53.1 Werz SM, Zeichner SJ, Berg BI, Zeilhofer HF, Thieringer F. 3D printed surgical simulation models as educational tool by maxillofacial surgeons. Eur J Dent Educ. 2018;22(3):e500–5. https://doi.org/10.1111/eje.12332.
- ↑ Legocki AT, Duffy-Peter A, Scott AR. Benefits and Limitations of Entry-Level 3-Dimensional Printing of Maxillofacial Skeletal Models. JAMA Otolaryngol Head Neck Surg. 2017;143(4):389–394. doi:10.1001/jamaoto.2016.3673
- ↑ Liu, K., Madbouly, S. A., Schrader, J. A., Kessler, M. R., Grewell, D. and Graves, W. R. (2015), Biorenewable polymer composites from tall oil-based polyamide and lignincellulose fiber. J. Appl. Polym. Sci., 132, 42592. doi: 10.1002/app.42592.
- ↑ Mills, C. A.; Navarro, M.; Engel, E.; Martinez, E.; Ginebra, M. P.; Planell, J.; Errachid, A.; Samitier, J. J. Biomed. Mater. Res. A 2006, 76A, 781.
- ↑ Auras, R.; Harte, B.; Selke, S. Macromol. Biosci. 2004, 4, 835.
- ↑ Husemoglu R. B., Baysan G., Ertugruluoglu P., Tuc Yucel A., Havitcioglu H. , The Mechanical Comparison of Artificial Bone and 3D Printed Bone Segments, Journal of Medical Innovation and Technology, 2020; 2 (2):127-130.
- ↑ Haffner M, Quinn A, Hsieh TY, Strong EB, Steele T. Optimization of 3D Print Material for the Recreation of Patient-Specific Temporal Bone Models. Ann Otol Rhinol Laryngol. 2018 May;127(5):338-343. doi: 10.1177/0003489418764987. PMID: 29667491.
- ↑ Staples Canada. Acme 4" Plastic Protractor [Internet]. Staples. 2021 [cited 2021 Dec 11]. Available from: https://www.staples.ca/products/38953-en-acme-4-plastic-protractor.
- ↑ Khokhotva M, Backstein D, Dubrowski A. Outcome errors are not necessary for learning orthopedic bone drilling. Can J Surg. 2009 Apr;52(2):98-102. PMID: 19399203; PMCID: PMC2663499. URL: https://pubmed.ncbi.nlm.nih.gov/19399203/.
- ↑ Wierinck E, Puttemans V, Swinnen S, van Steenberghe D. Effect of augmented visual feedback from a virtual reality simulation system on manual dexterity training. Eur J Dent Educ. 2005 Feb;9(1):10-6. doi: 10.1111/j.1600-0579.2004.00351.x. PMID: 15642018.