Scientific researchers have conducted countless studies on the control strategies of greenhouse microclimate. Different techniques, including optimizing the structural characteristics (e.g., orientation, geometry, covering material), applying various modern control algorithms (e.g., AI-based control systems), and utilizing passive or active energy management systems (e.g., geothermal heating/cooling systems, solar collectors, storage systems) have been assessed thoroughly in the literature to proffer the most sustainable greenhouse(s) compatible with each location’s circumstances (Achour et al., 2021; Badji et al., 2022; Choab et al., 2019)[1][2][3]. In the field of energy management, prior investigations have implemented diverse approaches to meet the heating/cooling demands of greenhouses (Choab et al., 2019)[3]. Regarding the environmental restrictions, especially in the upper latitudes, many researchers focus on sustainable energy sources/technologies such as solar and geothermal energy and heat pumps (Gorjian et al., 2021)[4]. This article explores the most significant studies on the application of heat pumps in covering the heating/cooling requirements of greenhouses by reviewing the following papers in the literature:
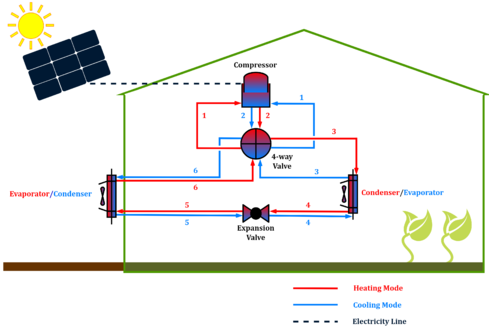
Economics of greenhouse heating with a mine air-assisted heat pump (S. Marsh & Singh, 1994)[5]
https://doi.org/10.13031/2013.28288
- Greenhouse heating system composed of a heat pump (source: an inactive deep mine) and a natural gas-fired heater as a backup system, located in Charleston, West Virginia.
- Without taking the capital costs into account, the heat pump had an economic advantage over the conventional one.
- Considering the investment costs, the life cycle cost of the heat pump was higher than the natural gas-fired heater.
- For the COP of above 3, the hybrid system becomes economically feasible.
Evaluation of the Feasibility of Alternative Energy Sources for Greenhouse Heating (Garcı́a et al., 1998)[6]
https://doi.org/10.1006/jaer.1997.0228
- Greenhouse heating systems (conventional fossil-fuel system and three hybrid systems, respectively composed of solar collectors, a heat pump, and a cogeneration system)-under the conditions of seven European locations.
- The heat pump and cogeneration system were more feasible in northern than the southern European climates.
- Solar flat plate collectors: not cost-effective
On the study of an energy-efficient greenhouse for heating, cooling and dehumidification applications (Chou et al., 2004)[7]
https://doi.org/10.1016/S0306-2619(03)00157-0
A heat pump with condenser and evaporator capacities of 30.0 kW and 37.0 kW is sufficient to maintain the indoor temperature of a greenhouse at 27 °C during the day and at 18 °C at night, and the indoor relative humidity around 40%.
The COP of this heat pump varied between 1.2-4.0.
An Economical Analysis on a Solar Greenhouse Integrated Solar Assisted Geothermal Heat Pump System (Ozgener & Hepbasli, 2005a)[8]
https://doi.org/10.1115/1.2126984
Experimental investigation of the performance of a solar-assisted ground-source heat pump system for greenhouse heating (Ozgener & Hepbasli, 2005b)[9]
https://doi.org/10.1002/er.1049
Performance analysis of a solar-assisted ground-source heat pump system for greenhouse heating: an experimental study (Ozgener & Hepbasli, 2005c)[10]
https://doi.org/10.1016/j.buildenv.2004.08.030
- Turkey: among the first five countries to exploit geothermal energy.
- Ground source heat pumps (GSHPs) have high investment costs compared to conventional split heating/cooling systems.
- The benefit/cost ratio of GSHPs is higher than that of the conventional systems in Turkey.
- The experimental results: the solar-assisted GSHP could be used for greenhouse heating in the Mediterranean and Aegean regions of Turkey.
Evaluation of a heat pump system for greenhouse heating (Aye et al., 2010)[11]
https://doi.org/10.1016/j.ijthermalsci.2009.07.002
- The viability of an air-to-water heat pump unit for a 4000 m2 greenhouse, located in Melbourne, Australia.
- Operating this heat pump could save up to 16% of LPG consumption in the conventional heater.
- The simple payback period of the heat pump: less than 6 years.
- The heat pump’s greenhouse gas emissions: 3% higher than those of the LPG boiler.
Energetic performance analysis of a ground-source heat pump system with latent heat storage for a greenhouse heating (Benli, 2011)[12]
https://doi.org/10.1016/j.enconman.2010.07.033
- A greenhouse integrated with a GSHP and latent heat thermal storage system.
- The COP of GSHP is higher than the conventional air source heat pumps (ASHPs).
- The compressor operation was relatively stable because the ground temperature was almost stable even during the coldest month.
- Put the heat pump inside the greenhouse or an insulated place in order to prevent the brine water from losing thermal energy on its path toward/from the ground.
- No need for an auxiliary heat source.
- The COP of the heat pump and the overall system: respectively in the ranges of 2.3-3.8 and 2-3.5.
Greenhouse heating using heat pumps with a high coefficient of performance (COP) (Tong et al., 2010)[13]
https://doi.org/10.1016/j.biosystemseng.2010.05.003
Greenhouse indoor temperature of 16 °C and the outside temperature within the range of -5 to 6 °C: the average hourly COP was 4.0 with a maximum value of 5.8.
Reductions in energy consumption and CO2 emissions for greenhouses heated with heat pumps (Tong et al., 2012)[14]
https://doi.org/10.13031/2013.41488
- Comparison of the performances of two heating systems (heat pump and kerosene heater) in two North-South oriented, single-span, saddle-roof greenhouses located in Kashiwa, Japan.
- For the ambient temperature of -5 to 6 °C, the hourly energy consumption in the greenhouse with the heat pump was about 0.22-0.56 MJ/m2.
- The same parameter was about 0.42-0.76 MJ/m2 for the greenhouse heated by the kerosene heater.
- The heat pump system: 1.3-2.6 times more efficient than the kerosene heater.
- The hourly CO2 emissions in the greenhouse with the heat pump: 9.5-24 g/m2.
- The same parameter was 31-55 g/m2 for the greenhouse heated by the kerosene heater.
- The heat pump system was cost-effective.
Performance evaluation of ground source heat pump system for greenhouse heating in northern China (Chai et al., 2012)[15]
https://doi.org/10.1016/j.biosystemseng.2011.11.002
- A Chinese style (G1) and a glass multi-span greenhouse (G2) located in China have been analyzed: technical, economic, and environmental.
- The average COP of the heat pump: 3.83 and 3.91, respectively, for G1 and G2 heating.
- The daily heating cost: 0.016 US$/m2d and 0.058 US$/m2d, respectively for G1 and G2.
- If the electricity demand of these heat pumps was provided by coal-fired power plants, the GSHP operation would increase the emissions of CO2 by 46.1% and 43.5% compared with the coal-fired heating system, respectively, in G1 and G2.
Experimental evaluation of using various renewable energy sources for heating a greenhouse (Esen & Yuksel, 2013)[16]
https://doi.org/10.1016/j.enbuild.2013.06.018
- A biogas production tank, solar flat plate collectors, and a GSHP with horizontal heat exchangers: to heat a greenhouse. This complex can be located in the East and Southeast regions of Turkey, where livestock manure, as biomass, must be available nearby.
- The GSHP, the biogas production tank, and also their combination: each can be a standalone greenhouse heating system.
- Solar energy system: can be a standalone heating system with high storage temperatures.
A performance comparison between a horizontal source and a vertical source heat pump systems for a greenhouse heating in the mild climate Elaziğ, Turkey (Benli, 2013)[17]
https://doi.org/10.1016/j.applthermaleng.2012.06.005
- The horizontal and vertical GSHPs, coupled with the greenhouses in which the seasonal products were being harvested.
- The COP of the heat pump: 3.1-3.6 and 3.2-3.6, respectively, for horizontal and vertical ones.
- The COP of the overall system: 2.7-3.3 and 2.9-3.5, respectively, for horizontal and vertical ones.
Evaluating the performance of a large borehole ground source heat pump for greenhouses in northern Japan (Li et al., 2013)[18]
https://doi.org/10.1016/j.energy.2013.09.009
- A GSHP coupled with 12 industrial greenhouses in Akabira, Japan.
- The heat pump and the whole system: heating COP of 3 and 2.7, respectively.
- The heat pump and the whole system: CO2 emissions of the system were 20% and 22% lower than that of the ASHP and kerosene systems, respectively.
Greenhouse Heating and Cooling with a Heat Pump System using Surplus Air and Underground Water Thermal Energy (S.-H. Yang et al., 2013)[19]
https://doi.org/10.1016/S1881-8366(13)80016-X
- The thermal energy of underground water and the surplus air rejected from the greenhouse were the heat sources of a heat pump system.
- A control system has also been implemented.
- The average COP: 3.25 and 2.84, respectively, in February and March.
Utilization and performance evaluation of a surplus air heat pump system for greenhouse cooling and heating (S.-H. Yang & Rhee, 2013)[20]
https://doi.org/10.1016/j.apenergy.2012.12.038
- The thermal energy of surplus air stream from a 100 m2 glass greenhouse to a heat pump and a thermal energy storage system, in Korea. Amount: between 258.3 and 6259.0 MJ/month.
- The mean monthly energy conservation: 0.63%, 10.36%, and 25.72%, respectively, in January, February, and March.
- Estimating the capital cost of the conservation system from the capital cost of the experimental setup: the payback period of the system 15.7 years if the system operate 5 months per year.
Development and evaluation of combustion-type CO2 enrichment system connected to heat pump for greenhouses (S.-H. Yang et al., 2014)[21]
https://doi.org/10.1016/j.eaef.2013.12.005
- A gas refinement to produce a toxic-free for greenhouse fertilization
- A thermal recovery system to utilize the thermal energy of CO2 gas stream to run a heat pump.
- A control algorithm for the effective operation of the proposed system.
- The mean heat recovery efficiency: 0.62, 0.87 and 1.18 for January, April and July, respectively.
Temperature distribution and performance of ground-coupled multi-heat pump systems for a greenhouse (Choi et al., 2014)[22]
https://doi.org/10.1016/j.renene.2013.07.010
- A ground-coupled multi-heat pump (GCHP) system consisting of a ground loop heat exchanger (GLHX) and multiple heat pumps.
- Installing multiple heat pump units inside the greenhouse minimizes the temperature fluctuations inside the greenhouse.
- It is required to develop a multi-heat pump unit that can operate with lower condenser temperatures, e.g., around 12 °C, to provide more thermal comfort for some crops, thereby increasing their yields.
Performance of the coupling of the flat plate collector and a heat pump system associated with a vertical heat exchanger for heating of the two types of greenhouses system (Awani et al., 2015)[23]
https://doi.org/10.1016/j.enconman.2015.06.032
For a solar-assisted GSHP integrated with a greenhouse in Tunisia: the drilling costs are a significant obstacle, and it is considerably related to the borehole depth.
Optimization of performance of Combined Solar Collector-Geothermal Heat Pump Systems to supply thermal load needed for heating greenhouses (Mehrpooya et al., 2015)[24]
https://doi.org/10.1016/j.enconman.2015.03.073
- The maximum COP without preheating the working fluid before the evaporator: 4.14.
- The simple payback period: 14 years.
Numerical modeling and economic analysis of a ground source heat pump for supplying energy for a greenhouse in Alborz province, Iran (Noorollahi et al., 2016)[25]
https://doi.org/10.1016/j.jclepro.2016.05.059
- A GSHP as a heating and air conditioning unit in a 1000 m2 greenhouse located in Karaj.
- The longer the ground heat exchanger, the higher the heat pump capacity.
- Using a GSHP in a greenhouse in Iran is not cost-effective since the capital cost of heat pumps and electricity prices are relatively high, and the price of natural gas is low.
Heat-pump dehumidifier as an efficient device to prevent condensation in horticultural greenhouses (Chantoiseau et al., 2016)[26]
https://doi.org/10.1016/j.biosystemseng.2015.11.011
- A heat pump reduces the relative humidity of greenhouse air in the evaporator and then heats up the dry air in the condenser and leads it into the greenhouse again.
- The heat pump-assisted dehumidification system consumed 6-8.5 times less energy than the conventional venting-heating system.
- This system was more cost-effective than conventional heating systems in several significant countries.
Operational energy saving potential of thermal effluent source heat pump system for greenhouse heating in jeju (Kim et al., 2017)[27]
https://doi.org/10.1142/S2010132517500304
- A heat recovery system and a heat pump, employed to supply three greenhouses with low, medium, and high-temperature crops, could respectively save 17%, 19%, and 20% of energy compared with the conventional GSHP.
Performance Assessment of Photovoltaic, Ground Source Heat Pump and Hydrogen Heat Generator in a Standalone Systems for Greenhouse Heating (A S Anifantis, 2017)[28]
https://doi.org/10.3303/CET1758086
Photovoltaic and Hydrogen Plant Integrated with a Gas Heat Pump for Greenhouse Heating: A Mathematical Study (Alexandros Sotirios Anifantis et al., 2018)[29]
https://doi.org/10.3390/su10020378
- Two PV-based GSHP systems were employed for greenhouse heating.
- First system: a PV system, an electrolyzer, a hydrogen storage system, a fuel cell, and a GSHP; Complicated and with 13% efficiency; Capable of increasing the greenhouse temperature 6-10 °C higher than the ambient temperature.
- Second system: analogous to the first one, but a direct hydrogen burner was embedded in the system instead of converting hydrogen in the fuel cell; Simple and with 7% efficiency; Capable of increasing the greenhouse temperature 3-7 °C higher than the ambient temperature.
Effect of ambient conditions on drying of herbs in solar greenhouse dryer with integrated heat pump (Tham et al., 2017)[30]
https://doi.org/10.1080/07373937.2016.1271984
- A low-temperature heat pump: a supplementary system for a solar dryer at night and on cloudy days.
- The desirable drying conditions could be maintained regardless of the ambient circumstances.
- The relative humidity (RH) of the drying room was reduced by 10-15 %.
- The drying rate of Java tea was enhanced up to fourfold.
- A 10% saving in drying time of both O-aristatus and C-nutans leaves.
- The consistency and uniformity of the drying temperature and room RH were guaranteed.
Numerical and experimental study of a closed loop for ground heat exchanger coupled with heat pump system and a solar collector for heating a glass greenhouse in north of Tunisia (Awani et al., 2017)[31]
https://doi.org/10.1016/j.ijrefrig.2017.01.030
- A horizontal GSHP assisted by a solar flat plate collector for greenhouse heating in Tunisia.
- The geothermal and solar-assisted heat pump was a promising alternative to conventional heating systems.
Evaluation of a hybrid system for a nearly zero energy greenhouse (Yildirim & Bilir, 2017)[32]
https://doi.org/10.1016/j.enconman.2017.06.068
- The near-zero energy greenhouse employing solar PV modules to power both a GSHP and provide lighting demands of three greenhouses with different crops, including tomato, cucumber, and lettuce.
- Because of the asymmetric design of the roof, 50% of the southern face was dedicated to placing PV panels.
- The seasonal COP of GSHP: 2.25 and 2.76, respectively, for cooling and heating.
- The electricity coverage ratio of solar PV panels for summer months: 33.2-67.2%.
- The electricity coverage ratio of solar PV panels for winter months: 95.7%, 86.8%, and 104.5%, respectively, for tomato, cucumber, and lettuce.
- The simple payback period for tomato, cucumber, and lettuce: 7.2, 7.4, and 7 years, respectively.
- The greenhouse gas payback period: 5.7 and 2.6 years, respectively, for natural gas and coal-based electricity generation.
The evacuated tube solar collector assisted heat pump for heating greenhouses (Hassanien et al., 2018)[33]
https://doi.org/10.1016/j.enbuild.2018.03.072
- An evacuated tube solar collector (ETC) for heating water, which was supplying a heat pump.
- The thermal efficiency and the payback period of ETC: 0.49 and 4.1 years.
- The COP of the heat pump: 4.24.
- It was not capable of meeting all the energy demands in January because of the considerable amount of heat losses from the hot water storage tank and the pipe connections.
Thermal performance of a conic basket heat exchanger coupled to a geothermal heat pump for greenhouse cooling under Tunisian climate (Boughanmi et al., 2015)[34]
https://doi.org/10.1016/j.enbuild.2015.07.004
A performance of a heat pump system connected a new conic helicoidal geothermal heat exchanger for a greenhouse heating in the north of Tunisia (Boughanmi et al., 2018)[35]
https://doi.org/https://doi.org/10.1016/j.solener.2018.06.054
- A novel conic helicoidal geothermal heat exchanger (CHGHE) has been connected to a heat pump to reduce the used area and the operating cost compared to horizontal and vertical heat exchangers.
- This heat pump system had a ceiling unit composed of a heat exchanger suspended in the air.
- Other heat exchangers were buried under the floor inside the greenhouse.
- As a result, they could provide 3.6 MJ of heat for the greenhouse at night; they ensured the indoor temperature rise of 3 °C and a relative humidity decrease of 6%.
- COP of the heat pump and the overall system: 3.9 and 2.82, respectively.
Analysis of the Possibilities of Using a Heat Pump for Greenhouse Heating in Polish Climatic Conditions—A Case Study (Nemś et al., 2018)[36]
https://doi.org/10.3390/su10103483
- An ASHP, a GSHP, and a conventional boiler in a greenhouse have been compared under Polish climatic conditions. Technical, Economic, and Environmental.
- COP for ASHP and GSHP with almost similar capacity: 2.59-4 and 5.53-5.83, respectively.
- The investment costs of GSHP, ASHP, and boiler: €34/m2, €12/m2, and €7.4/m2, respectively.
- The current electricity costs of GSHP and ASHP: €385.60/year and €678.64/year, respectively.
- The payback period of GSHP and ASHP: 18 and 5.5 years, respectively.
- The annual profit earned from operating the GSHP and existing boiler: 62%.
- The annual profit earned from operating the ASHP: 32%.
Heating performance analysis of an air-to-water heat pump using underground air for greenhouse farming (Lim et al., 2020)[37]
https://doi.org/10.3390/en13153863
- The exploitation of the underground air as a heat source for a water-to-water heat pump heating a greenhouse located in South Korea. The underground air can not be directly used because of its high humidity and low temperature.
- A specific auxiliary heat exchanger designed to heat up the water by underground air.
- The COP of the proposed heat pump: 2.1-2.7 for providing 34.9-44.2 kW heating.
- This system saved 75% of the total heating costs imposed by the conventional heater.
- The closed-loop version had a COP smaller by 50%.
Thermo-economic comparison of coal-fired boiler-based and groundwater-heat-pump based heating and cooling solution – A case study on a greenhouse in Hubei, China (Luo et al., 2020)[38]
https://doi.org/10.1016/j.enbuild.2020.110214
- Replacing a conventional boiler heater with a GSHP for heating and cooling in a greenhouse.
- The heat pump is a better choice than a boiler because a heat pump also plays the role of an air conditioner in the summer.
- The capital and operation costs of GSHP: higher than that of the coal-fired boiler.
- The Average Energy Price (AEP) of GSHP will be reduced during the ten years. In contrast, the AEP of the boiler will be increased.
An experimental analysis of a solar-assisted heat pump (SAHP) system for heating a semisolar greenhouse (Hematian et al., 2021)[39]
https://doi.org/10.1080/15567036.2019.1663308
- An experimental study on a SAHP for heating a greenhouse located in Tabriz (Northwest of Iran).
- Any increase in the solar irradiance and the ambient temperature, and a decrement in the tank temperature and compressor speed, caused an enhancement in the COP of the heat pump.
- An increase in wind speed improved the thermal performance of the system and reduced the collector efficiency. However, its effect on the performance of the system is not considerable compared to the effect of other factors.
Comparative performance analysis of a solar assisted heat pump for greenhouse heating in Tunisia (Agrebi et al., 2021)[40]
https://doi.org/10.1016/j.ijrefrig.2021.06.004
- A solar-assisted heat pump was heating a bench-scale greenhouse in Tunisia.
- The COP of the heat pump was directly related to the water temperature at the evaporator outlet.
- The flat plate collector helps in extending the lifetime of the heat pump and decreasing the electrical energy consumption by 18%.
Energy saving strategies in sustainable greenhouse cultivation in the mediterranean climate – A case study (Ouazzani Chahidi et al., 2021)[41]
https://doi.org/10.1016/j.apenergy.2020.116156
- The semi-transparent PV panels on the roof, a passive cooling system (natural ventilation and shading system), and a GSHP have been studied from the viewpoint of energy-saving potentials in a greenhouse in Italy.
- Implementing the EnergyPlus: the operable windows could save 16% energy consumption in greenhouse cooling.
- The heat pump could save 21% of primary energy, compared to the gas boiler and chiller.
- The semi-transparent PV panels supplied 16% and 44% of the annual electrical demand and the annual air conditioning electrical needs, respectively.
Experimental investigation of a ground-source heat pump system for greenhouse heating–cooling (Harjunowibowo et al., 2021)[42]
https://doi.org/10.1093/ijlct/ctab052
- A novel borehole soil-based thermal energy storage consisting 24 single vertical type heat exchangers integrated with horizontal tubes: to store the produced heat in the summer and the transitional seasons; serve as a supplementary heating source in the winter.
- An insulating foam: to reduce the thermal losses of the ground heat exchanger as much as possible.
- The PV panels were installed on the walls of a 46.94 m2 greenhouse, located in UK.
- Semi-transparent PV panels were mounted on the roof: to power the GSHP.
- The soil-based thermal storage and insulation system reduced the soil-thermal imbalance ratio by 33%, annually.
- The heating and cooling COP of the heat pump: 1.48–2.97 and 1.20–3.45, respectively.
Ground source heat pump (GSHP) systems for horticulture greenhouses adjacent to highway interchanges: A case study in South Korea (Seo & Seo, 2021)[43]
https://doi.org/10.1016/j.rser.2020.110194
- A GSHP as the core component of a greenhouse heating system: installed in greenhouses near highway interchanges and junctions in South Korea.
- The COP of the testbed: of 3.4-3.6; the simulated COP: 3.7.
- Four predefined scenarios: 1) heating with GSHP instead of oil boiler, 2) heating and cooling with GSHP replacing the oil boiler and air conditioner units, 3) heating and cooling with GSHP running at full capacity, and 4) heating with GSHP ensuring a fixed profit margin.
- The 3rd scenario with the highest financial merit.
- The 1st and the 2nd scenarios with the worst outcomes because the gained profit was not sufficient to make up for the operation costs.
- The gap between the capital and operation costs of heat pumps was larger than that of the oil boilers: Accordingly, the heat pump systems can save much more energy than the conventional systems.
Development and validation of air-to-water heat pump model for greenhouse heating (Rasheed et al., 2021)[44]
https://doi.org/10.3390/en14154714
Modeling-based energy performance assessment and validation of air-to-water heat pump system integrated with multi-span greenhouse on cooling mode (Rasheed et al., 2022)[45]
https://doi.org/10.3390/agronomy12061374
- An air-to-water heat pump (AWHP) for cooling purposes in a three-span greenhouse.
- The greenhouse thermal model using TRNSYS.
- The refrigerant of the heat pump was R410A.
- An increase in the ambient temperature led to an increase in the power consumed by the AWHP.
- The maximum energy demand in the greenhouse with an indoor temperature of 25 °C: 0.4 kW/m2; the heat pump provided 0.2 kW/m2 of it.
Demonstration study on ground source heat pump heating system with solar thermal energy storage for greenhouse heating (X. Yang et al., 2022)[46]
https://doi.org/10.1016/j.est.2022.105298
- A seasonal solar thermal energy storage (SSTES) + a diurnal solar thermal energy storage (DSTES) were assisting a GSHP in a greenhouse in China.
- The COP of GSHP without DSTES was 2.79.
- In the cold season: the DSTES reduced the GSHP electricity consumption and covered the power consumption of SSTES: Thus, it increased the annual COP to 3.19.
- Implanting long ground heat exchanger tubes could also save excess expenses by reducing the capacity of GSHP.
- Adjusting the temperature of SSTES on an appropriate level: shortening the operation time of circulating pumps + reducing the electric energy consumption rate.
Greenhouse heating by energy transfer between greenhouses: System design and implementation (Sun et al., 2022)[47]
https://doi.org/10.1016/j.apenergy.2022.119815
- The surplus air heat of Chinese-style greenhouse (CSG) + ambient air: two sources of a heat pump for a multi-span greenhouse in China.
- Utilizing the CSG surplus air heat: the heat pump’s COP could reach 3.4-4.2, 23-26% higher than when ambient air was connected to the evaporator.
- This heat pump could switch the heat sources. So, its COP was 6-11% higher than conventional ASHP.
- The minimum area of CSG must be twice the area of the multi-span greenhouse.
Meeting the electricity demand for the heating of greenhouses with hydrogen: Solar photovoltaic-hydrogen-heat pump system application in Turkey (Özçelep et al., 2023)[48]
https://doi.org/10.1016/j.ijhydene.2022.10.125
- Hydrogen and solar PV panels are used to power the heat pump in a greenhouse during the whole year.
- Neither hydrogen nor heat pump can meet the whole thermal demand of large-scale greenhouses.
- Integrating hydrogen energy and a heat pump system into a 24 m2 solar PV array will provide the heating demand of a 1000 m2 greenhouse in Bismil, Turkey.
References[edit | edit source]
- ↑ Achour, Y., Ouammi, A., & Zejli, D. (2021). Technological progresses in modern sustainable greenhouses cultivation as the path towards precision agriculture. Renewable and Sustainable Energy Reviews, 147, 111251. https://doi.org/https://doi.org/10.1016/j.rser.2021.111251
- ↑ Badji, A., Benseddik, A., Bensaha, H., Boukhelifa, A., & Hasrane, I. (2022). Design, technology, and management of greenhouse: A review. Journal of Cleaner Production, 373, 133753. https://doi.org/https://doi.org/10.1016/j.jclepro.2022.133753
- ↑ 3.0 3.1 Choab, N., Allouhi, A., El Maakoul, A., Kousksou, T., Saadeddine, S., & Jamil, A. (2019). Review on greenhouse microclimate and application: Design parameters, thermal modeling and simulation, climate controlling technologies. Solar Energy, 191, 109–137. https://doi.org/https://doi.org/10.1016/j.solener.2019.08.042
- ↑ Gorjian, S., Ebadi, H., Najafi, G., Singh Chandel, S., & Yildizhan, H. (2021). Recent advances in net-zero energy greenhouses and adapted thermal energy storage systems. Sustainable Energy Technologies and Assessments, 43, 100940. https://doi.org/https://doi.org/10.1016/j.seta.2020.100940
- ↑ S. Marsh, L., & Singh, S. (1994). Economics of Greenhouse Heating with a Mine Air-assisted Heat Pump. Transactions of the ASAE, 37(6), 1959–1963. https://doi.org/https://doi.org/10.13031/2013.28288
- ↑ Garcı́a, J. L., De la Plaza, S., Navas, L. M., Benavente, R. M., & Luna, L. (1998). Evaluation of the Feasibility of Alternative Energy Sources for Greenhouse Heating. Journal of Agricultural Engineering Research, 69(2), 107–114. https://doi.org/https://doi.org/10.1006/jaer.1997.0228
- ↑ Chou, S. K., Chua, K. J., Ho, J. C., & Ooi, C. L. (2004). On the study of an energy-efficient greenhouse for heating, cooling and dehumidification applications. Applied Energy, 77(4), 355–373. https://doi.org/https://doi.org/10.1016/S0306-2619(03)00157-0
- ↑ Ozgener, O., & Hepbasli, A. (2005a). An Economical Analysis on a Solar Greenhouse Integrated Solar Assisted Geothermal Heat Pump System. Journal of Energy Resources Technology, 128(1), 28–34. https://doi.org/10.1115/1.2126984
- ↑ Ozgener, O., & Hepbasli, A. (2005b). Experimental investigation of the performance of a solar-assisted ground-source heat pump system for greenhouse heating. International Journal of Energy Research, 29(3), 217–231. https://doi.org/https://doi.org/10.1002/er.1049
- ↑ Ozgener, O., & Hepbasli, A. (2005c). Performance analysis of a solar-assisted ground-source heat pump system for greenhouse heating: an experimental study. Building and Environment, 40(8), 1040–1050. https://doi.org/https://doi.org/10.1016/j.buildenv.2004.08.030
- ↑ Aye, L., Fuller, R. J., & Canal, A. (2010). Evaluation of a heat pump system for greenhouse heating. International Journal of Thermal Sciences, 49(1), 202–208. https://doi.org/https://doi.org/10.1016/j.ijthermalsci.2009.07.002
- ↑ Benli, H. (2011). Energetic performance analysis of a ground-source heat pump system with latent heat storage for a greenhouse heating. Energy Conversion and Management, 52(1), 581–589. https://doi.org/https://doi.org/10.1016/j.enconman.2010.07.033
- ↑ Tong, Y., Kozai, T., Nishioka, N., & Ohyama, K. (2010). Greenhouse heating using heat pumps with a high coefficient of performance (COP). Biosystems Engineering, 106(4), 405–411. https://doi.org/https://doi.org/10.1016/j.biosystemseng.2010.05.003
- ↑ Tong, Y., Kozai, T., Nishioka, N., & Ohyama, K. (2012). Reductions in Energy Consumption and CO2 Emissions for Greenhouses Heated with Heat Pumps. Applied Engineering in Agriculture, 28(3), 401–406. https://doi.org/https://doi.org/10.13031/2013.41488
- ↑ Chai, L., Ma, C., & Ni, J.-Q. (2012). Performance evaluation of ground source heat pump system for greenhouse heating in northern China. Biosystems Engineering, 111(1), 107–117. https://doi.org/https://doi.org/10.1016/j.biosystemseng.2011.11.002
- ↑ Esen, M., & Yuksel, T. (2013). Experimental evaluation of using various renewable energy sources for heating a greenhouse. Energy and Buildings, 65, 340–351. https://doi.org/https://doi.org/10.1016/j.enbuild.2013.06.018
- ↑ Benli, H. (2013). A performance comparison between a horizontal source and a vertical source heat pump systems for a greenhouse heating in the mild climate Elaziğ, Turkey. Applied Thermal Engineering, 50(1), 197–206.
- ↑ Li, H., Nagano, K., Lai, Y., Shibata, K., & Fujii, H. (2013). Evaluating the performance of a large borehole ground source heat pump for greenhouses in northern Japan. Energy, 63, 387–399. https://doi.org/https://doi.org/10.1016/j.energy.2013.09.009
- ↑ Yang, S.-H., Lee, S.-D., Kim, Y. J., & Rhee, J. Y. (2013). Greenhouse Heating and Cooling with a Heat Pump System using Surplus Air and Underground Water Thermal Energy. Engineering in Agriculture, Environment and Food, 6(3), 86–91. https://doi.org/https://doi.org/10.1016/S1881-8366(13)80016-X
- ↑ Yang, S.-H., & Rhee, J. Y. (2013). Utilization and performance evaluation of a surplus air heat pump system for greenhouse cooling and heating. Applied Energy, 105, 244–251. https://doi.org/https://doi.org/10.1016/j.apenergy.2012.12.038
- ↑ Yang, S.-H., Lee, C. G., Ashtiani-Araghi, A., Kim, J. Y., & Rhee, J. Y. (2014). Development and evaluation of combustion-type CO2 enrichment system connected to heat pump for greenhouses. Engineering in Agriculture, Environment and Food, 7(1), 28–33. https://doi.org/https://doi.org/10.1016/j.eaef.2013.12.005
- ↑ Choi, J. M., Park, Y.-J., & Kang, S.-H. (2014). Temperature distribution and performance of ground-coupled multi-heat pump systems for a greenhouse. Renewable Energy, 65, 49–55. https://doi.org/https://doi.org/10.1016/j.renene.2013.07.010
- ↑ Awani, S., Chargui, R., Kooli, S., Farhat, A., & Guizani, A. (2015). Performance of the coupling of the flat plate collector and a heat pump system associated with a vertical heat exchanger for heating of the two types of greenhouses system. Energy Conversion and Management, 103, 266–275. https://doi.org/https://doi.org/10.1016/j.enconman.2015.06.032
- ↑ Mehrpooya, M., Hemmatabady, H., & Ahmadi, M. H. (2015). Optimization of performance of Combined Solar Collector-Geothermal Heat Pump Systems to supply thermal load needed for heating greenhouses. Energy Conversion and Management, 97, 382–392. https://doi.org/https://doi.org/10.1016/j.enconman.2015.03.073
- ↑ Noorollahi, Y., Bigdelou, P., Pourfayaz, F., & Yousefi, H. (2016). Numerical modeling and economic analysis of a ground source heat pump for supplying energy for a greenhouse in Alborz province, Iran. Journal of Cleaner Production, 131, 145–154. https://doi.org/https://doi.org/10.1016/j.jclepro.2016.05.059
- ↑ Chantoiseau, E., Migeon, C., Chasseriaux, G., & Bournet, P.-E. (2016). Heat-pump dehumidifier as an efficient device to prevent condensation in horticultural greenhouses. Biosystems Engineering, 142, 27–41. https://doi.org/https://doi.org/10.1016/j.biosystemseng.2015.11.011
- ↑ Kim, M.-H., Lee, D.-W., Yun, R., & Heo, J. (2017). Operational Energy Saving Potential of Thermal Effluent Source Heat Pump System for Greenhouse Heating in Jeju. International Journal of Air-Conditioning and Refrigeration, 25(04), 1750030. https://doi.org/10.1142/S2010132517500304
- ↑ Anifantis, A S. (2017). Performance Assessment of Photovoltaic, Ground Source Heat Pump and Hydrogen Heat Generator in a Stand-Alone Systems for Greenhouse Heating . Chemical Engineering Transactions, 58, 511-516 SE-Research Articles. https://doi.org/10.3303/CET1758086
- ↑ Anifantis, Alexandros Sotirios, Colantoni, A., Pascuzzi, S., & Santoro, F. (2018). Photovoltaic and Hydrogen Plant Integrated with a Gas Heat Pump for Greenhouse Heating: A Mathematical Study. Sustainability, 10(2). https://doi.org/10.3390/su10020378
- ↑ Tham, T. C., Ng, M. X., Gan, S. H., Chua, L. S., Aziz, R., Chuah, L. A., Hii, C. L., Ong, S. P., Chin, N. L., & Law, C. L. (2017). Effect of ambient conditions on drying of herbs in solar greenhouse dryer with integrated heat pump. Drying Technology, 35(14), 1721–1732. https://doi.org/10.1080/07373937.2016.1271984
- ↑ Awani, S., Kooli, S., Chargui, R., & Guizani, A. (2017). Numerical and experimental study of a closed loop for ground heat exchanger coupled with heat pump system and a solar collector for heating a glass greenhouse in north of Tunisia. International Journal of Refrigeration, 76, 328–341. https://doi.org/https://doi.org/10.1016/j.ijrefrig.2017.01.030
- ↑ Yildirim, N., & Bilir, L. (2017). Evaluation of a hybrid system for a nearly zero energy greenhouse. Energy Conversion and Management, 148, 1278–1290. https://doi.org/https://doi.org/10.1016/j.enconman.2017.06.068
- ↑ Hassanien, R. H. E., Li, M., & Tang, Y. (2018). The evacuated tube solar collector assisted heat pump for heating greenhouses. Energy and Buildings, 169, 305–318. https://doi.org/https://doi.org/10.1016/j.enbuild.2018.03.072
- ↑ Boughanmi, H., Lazaar, M., Bouadila, S., & Farhat, A. (2015). Thermal performance of a conic basket heat exchanger coupled to a geothermal heat pump for greenhouse cooling under Tunisian climate. Energy and Buildings, 104, 87–96. https://doi.org/https://doi.org/10.1016/j.enbuild.2015.07.004
- ↑ Boughanmi, H., Lazaar, M., & Guizani, A. (2018). A performance of a heat pump system connected a new conic helicoidal geothermal heat exchanger for a greenhouse heating in the north of Tunisia. Solar Energy, 171, 343–353. https://doi.org/https://doi.org/10.1016/j.solener.2018.06.054
- ↑ Nemś, A., Nemś, M., & Świder, K. (2018). Analysis of the Possibilities of Using a Heat Pump for Greenhouse Heating in Polish Climatic Conditions—A Case Study. In Sustainability (Vol. 10, Issue 10). https://doi.org/10.3390/su10103483
- ↑ Lim, T., Baik, Y.-K., & Kim, D. D. (2020). Heating Performance Analysis of an Air-to-Water Heat Pump Using Underground Air for Greenhouse Farming. Energies, 13(15). https://doi.org/10.3390/en13153863
- ↑ Luo, J., Xue, W., & Shao, H. (2020). Thermo-economic comparison of coal-fired boiler-based and groundwater-heat-pump based heating and cooling solution – A case study on a greenhouse in Hubei, China. Energy and Buildings, 223, 110214. https://doi.org/https://doi.org/10.1016/j.enbuild.2020.110214
- ↑ Hematian, A., Ajabshirchi, Y., Ranjbar, S. F., & Taki, M. (2021). An experimental analysis of a solar-assisted heat pump (SAHP) system for heating a semisolar greenhouse. Energy Sources, Part A: Recovery, Utilization, and Environmental Effects, 43(14), 1724–1744. https://doi.org/10.1080/15567036.2019.1663308
- ↑ Agrebi, S., Chargui, R., Tashtoush, B., & Guizani, A. (2021). Comparative performance analysis of a solar assisted heat pump for greenhouse heating in Tunisia. International Journal of Refrigeration, 131, 547–558. https://doi.org/https://doi.org/10.1016/j.ijrefrig.2021.06.004
- ↑ Ouazzani Chahidi, L., Fossa, M., Priarone, A., & Mechaqrane, A. (2021). Energy saving strategies in sustainable greenhouse cultivation in the mediterranean climate – A case study. Applied Energy, 282, 116156. https://doi.org/https://doi.org/10.1016/j.apenergy.2020.116156
- ↑ Harjunowibowo, D., Omer, S. A., & Riffat, S. B. (2021). Experimental investigation of a ground-source heat pump system for greenhouse heating–cooling. International Journal of Low-Carbon Technologies, 16(4), 1529–1541. https://doi.org/10.1093/ijlct/ctab052
- ↑ Seo, Y., & Seo, U.-J. (2021). Ground source heat pump (GSHP) systems for horticulture greenhouses adjacent to highway interchanges: A case study in South Korea. Renewable and Sustainable Energy Reviews, 135, 110194. https://doi.org/https://doi.org/10.1016/j.rser.2020.110194
- ↑ Rasheed, A., Kim, H. T., & Lee, H. W. (2022). Modeling-Based Energy Performance Assessment and Validation of Air-To-Water Heat Pump System Integrated with Multi-Span Greenhouse on Cooling Mode. Agronomy, 12(6). https://doi.org/10.3390/agronomy12061374
- ↑ Rasheed, A., Na, W. H., Lee, J. W., Kim, H. T., & Lee, H. W. (2021). Development and Validation of Air-to-Water Heat Pump Model for Greenhouse Heating. Energies, 14(15). https://doi.org/10.3390/en14154714
- ↑ Yang, X., Sun, D., Li, J., Yu, C., Deng, Y., & Yu, B. (2022). Demonstration study on ground source heat pump heating system with solar thermal energy storage for greenhouse heating. Journal of Energy Storage, 54, 105298. https://doi.org/https://doi.org/10.1016/j.est.2022.105298
- ↑ Sun, W., Wei, X., Zhou, B., Lu, C., & Guo, W. (2022). Greenhouse heating by energy transfer between greenhouses: System design and implementation. Applied Energy, 325, 119815. https://doi.org/https://doi.org/10.1016/j.apenergy.2022.119815
- ↑ Özçelep, Y., Bekdaş, G., & Apak, S. (2023). Meeting the electricity demand for the heating of greenhouses with hydrogen: Solar photovoltaic-hydrogen-heat pump system application in Turkey. International Journal of Hydrogen Energy, 48(7), 2510–2517. https://doi.org/https://doi.org/10.1016/j.ijhydene.2022.10.125