
The need for renewable energy technologies has become apparent and is continuously gaining speed, particularly due to increasing concerns about climate change, pollution, and predicted non-affordable costs related to fossil fuels consumption[1][2]. Among the renewable energy sources (RESs), solar and wind energy have gained much more attention in electricity generation since 2008.[2] However, not only does the absolute dependence on solar and wind energy have some technical challenges, but they can not afford the whole energy demands of the world. It is worth noting that the overall primary energy use was expected to rise from 550 EJ (EJ=exajoule=1018 joule) in 2015 to 720 EJ in 2035, let alone all RESs were forecast to roughly double from their 2015 level of 52.7 EJ to 119.6 EJ in 2035.[2]
Nima Asgari, an independent researcher in the field of renewable energies, has raised a proposal to address the challenges of solar energy (especially photovoltaics) harnessing without taking advantage of other RESs and other supplementary technologies. He has presented the pros and cons of solar energy utilizing and then has illustrated the whole picture of all possible supplementary technologies whose simultaneous use with solar energy provides promising benefits for humans. Eventually, he has defined the methodology of the whole project by dividing it into several analytical steps.
Solar Energy[edit | edit source]
Solar energy means energy from sunlight in any form[3], and there is no doubt that solar energy gives life to human beings and all living organisms on the planet while causing all the natural phenomena, including winds, sea tides, and seasonal fluctuations. Therefore, the Sun is responsible for all renewable energy sources on Earth, which meet the needs of human beings.[4]
Applications[edit | edit source]

A partial list of solar applications includes space heating and cooling through solar architecture, daylighting, solar hot water (Fig. 1[3]), solar cooking, distillation, disinfection, and high-temperature process heat for industrial purposes like power generation. The most common way of solar energy harvesting is to use solar panels.
Plus points[3][edit | edit source]
The solar energy benefits are multiple:
- Solar energy is one of the primary renewable energy sources.
- A solar system can substantially reduce electricity bills.
- Solar energy can be used for different purposes, such as electricity (photovoltaics) and heat (solar thermal).
- Solar energy systems generally require low maintenance costs.
- The technological developments taking place within the solar panel industry assure that solar panels will continue being one of the most effective sustainable energy sources on the planet.
Challenges[edit | edit source]
- Although the position of the Sun in the sky follows a fairly predictable pattern, in addition to its seasonal fluctuations, some other climatic circumstances affect the quality and the quantity of solar energy we can obtain. The other problem is with its consumption patterns: the sudden spikes in demand (a smaller one in the morning and the larger peak as the Sun sets) do not correspond with heightened solar energy generation. Simply put, the supply and demand charts are mismatched[5]. The increasing application of renewable energy technologies, especially solar and wind energy, to feed into power grids is a challenge to the system operators, who must then deal with more unpredictable net loads and more complex balancing[6].
- If used for 20 to 25 years, as their warranty suggests, solar panels can be a very clean form of energy. A solar cell is made up of silicon, which is made by heating quartz to extremely high temperatures (1200 Celsius), releasing a lot of carbon emissions in the process. Additionally, turning metallurgical-grade silicon into a purer form called polysilicon creates the very toxic compound silicon tetrachloride. Fortunately, there is a process that most manufacturers employ to safely recycle silicon tetrachloride back into the manufacturing process for new silicon wafers, helping to eliminate health and environmental risks..[5]
- Utility-grade solar plants require disproportionately large areas to house enough solar panels (or mirrors, in the case of concentrated solar).[5]
Hybridization[edit | edit source]
Despite these issues, solar energy is still a uniquely useful source of renewable energy: plentiful, cheap to maintain, and clean. So, how to overcome the limitations of solar energy?
Scientists have developed various advanced technologies for energy exploitation and transferring with characteristics compatible with solar energy technologies, in recent decades. The most appropriate and rational strategy is the hybridization of solar energy technologies with supplementary renewable energy sources, energy storage systems (ESSs), hydrogen technology, and multi-generation systems.
Supplementary renewable energy sources (RESs)[edit | edit source]

A hybrid system of solar technology and other renewable-based supply sources is highly recommended to address the unpredictability of achievable solar energy. Since the direct vertical sunlight is meager in some northern latitudes, solar energy is not a reliable source to meet humans' demands, especially in the cold periods. In some areas like Iceland (Fig. 2[7]), Northern Iran, and Northern California, owing to the volcanic activities and geological conditions, the geothermal energy potentials are indisputable choices to be combined with solar energy[8][9]. Furthermore, in some multi-storey and high-rise buildings, engineers encounter the lack of available land to provide all the electricity and heating/cooling requirements of the residents. In these cases, with a special focus on the buildings' wastewater and solid wastes, the biomass resources can be involved in addressing the problem. Consequently, the development of combined renewable systems stabilizes production costs by reducing the unpredictable impact of solely solar energy exploitation.
Some of these combined renewable energy technologies are small distributed electricity generation facilities for on-site use (e. g., rooftop solar system). On the other hand, some of them are community-scale distributed electricity generation systems whose power is not used on-site but rather flows onto the distribution grid.[10]
Energy storage systems (ESSs)[edit | edit source]
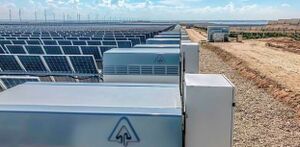
There are different types of energy storage technologies, including pumped hydro energy storage (PHES), thermal energy storage (TES), flywheel energy storage, compressed air energy storage (CAES), thermochemical energy storage (TCES), different types of batteries (Fig. 3[11]), etc.[12]. Each one has different technical qualities, costs, and lifetimes[13], which made them flexible in application with the solar technologies (e. g., concentrated solar power (CSP), solar photovoltaic/thermal (PV, PVT), and flat plate collector (FPC)) to bridge the gap between the supply and consumption patterns in buildings and industrial sectors. Energy storage systems capture energy in the form of thermal energy, electricity, or mechanical energy when the supply level is higher than the demand level, and release it when the conditions are reversed[14]. Thus, flexibility of solar technologies can be achieved only when the generation and the demand side become flexible with storage systems acting as the mediators managing their interconnection[15].
Hydrogen technology[edit | edit source]
Solar-based hydrogen generation is a reliable alternative to conventional solutions dealing with the use of fossil fuels in hydrogen production, which involves the emission of greenhouse gases and other pollutants. Therefore, the focus of researchers is on the integration of solar hydrogen technologies in hybrid and innovative systems. In particular, four main types of solar-based hydrogen generation technologies can be identified: PV, solar thermal and thermochemical, photoelectrolysis, and biophotolysis[16]. Some of these processes like PV and CSP may be used for producing electricity, which can be utilized by an electrolyzer in order to produce hydrogen[16][17], and the other ones like thermochemical cycles and photoelectrolysis can be considered as the direct form of hydrogen production from solar energy. Needles to say, the produced hydrogen not only makes up for the economic drawbacks of solar facilities applications on a large scale but also provides a clean chemical source for auxiliary power generation by fuel cells when the consumption level reaches the peak points.
Multi-generation systems[edit | edit source]


In general, about 24% of the total global energy consumption is merely contributed to buildings' heating, ventilation, air conditioning, and cooling (HVAC) systems[18][19], which reveals the great importance of these systems in energy management. Besides, some forms of solar energy power suppliers, such as CSP plants, are commonly established in arid areas, which suffer from water scarcity (Fig. 4 & 5[20])[21]. Addressing these issues, multi-generation systems (i. e., the combined production of power, heating, cooling, fuel, fresh water, etc.) can also help the balancing, efficiency-improvement, and economic feasibility of solar systems[22]. Therefore, employing solar-based multi-generation systems for providing heating and cooling demands (using both the 4th and 5th generation heating/cooling technologies), along with driving desalination systems (e. g., humidification-dehumidification (HDH), Reverse Osmosis (RO), and Multi-effect distillation (MED)) is promising.
The challenges should be addressed![edit | edit source]
Reviewing the literature reveals that there are plentiful studies on hybrid applications of solar energy with other technologies in power generation. However, there are still remaining questions for researchers: What are the most recent advancements in solar hybrids? What are the advantages and disadvantages of each hybrid? What are the scientific gaps in solar hybrids? Which countries (or climates) are suitable for each hybrid? Which hybrid configuration has the off-grid use potentials?
Some hybrid technologies of hydrogen production and ESSs have high investment costs, service time, operating and maintenance costs. Also, power transmission and distribution costs should be investigated for community-scale power plants. In addition, the environmental impacts of biomass fuels conversion considering their carbon cycle must be studied. Simultaneously, questions like what are the environmental benefits of such hybrids and which hybrid is more environmentally friendly should be answered. Energy, the environment, and the economic development of a country are closely related. The proper use of energy requires consideration of social impacts as well as technological ones. Indeed, the sustainable economic growth of a country may be possible only by the well-planned and efficient use of locally available renewable resources such as solar energy, wind, hydro, and biomass.[4] Hence, the viability, economic feasibility, and reliability analyses of hybrid systems give a framework for the implementation of further sustainability analysis, and accordingly, alongside the energy, environmental, and economic investigations, the sustainability assessment employing analytical tools, including but not limited to Life Cycle Assessment (LCA), Environmental Risk Assessment, and System Dynamics should be accomplished.[23]
Optimization can be used to find the best solar hybrid configurations considering the above-mentioned objectives. In this regard, the questions are: What are the optimal arrangement and design conditions of each hybrid? Which hybrid has the lowest payback time and the highest profit in its service time? Are there different ideal hybrids from the economic aspect? Is there a single ideal condition from both the economic and performance points of view? The answer to these questions can be merely provided by defining multi-objective optimization problems under the variation of numerous variables, which makes engineers employ complex mathematical models and high-tech computers.
Methodology[edit | edit source]
In general, the methods are based on simulation, ranging from technical modeling to optimization. The objectives of the present project will be fulfilled by six work packages (WPs), each directly addressing the above-raised concerns.
WP1 (Detailed Literature Review)[edit | edit source]
In the first step, a comprehensive study must be carried out in literature to recognize the most recent advancements in the related areas and define the technological preferences based on the researchers' authentic results and conclusions: What kind of renewable energies have the most compatibility with the solar technologies? What are the most advanced technologies in exploiting each renewable energy source for different aims and from different viewpoints? What are the most advanced auxiliary equipment or technologies like energy storage systems, heat exchangers, hydrogen storages, turbomachines, valves, separators, vessels, connectors, etc.? Answering all these general questions will limit the researchers' preferences, thereby optimizing their efforts. At this point, the existing research gaps should also be detected with the aim of solving them in the following steps. Needless to say, several Review articles can come out based on the results of these studies.
WP2 (Preliminary Modeling Based on the Experimental Investigations)[edit | edit source]
The first step's outcomes would help researchers to offer diverse configurations of hybrid renewable-based power and multi-generation systems employing various energy storage systems. In order to account for the particular advantages and disadvantages of each hybrid system, the energy and exergy modeling would be implemented for all these configurations, and these modelings must be validated based on the experimental investigations of different energy harvesting technologies such as solar PVs, biomass digestion, heat pumps, etc. It is highly recommended that the experimental assessments take place by the researchers involved in this project to obtain more reliable results under the provided circumstances. A parametric analysis will also be done to identify the critical parameters of each system. Therefore, the least efficient components would be replaced by the better alternatives, and the other distinct energetic and exergetic deficiencies should be paid attention to. All these modifications should be done merely on the general composition of the proposed systems since further studies have not been done considering the economic and environmental objectives.
WP3 (Advanced Economic and Environmental Modeling)[edit | edit source]
Since complex systems need high investment costs, precise economic analysis should be considered for their successful deployment. At the first level, the reliability assessment will be combined with the Markov method in thermo-economics to scrutinize the cost variations of the products for achieving a more robust cost estimation. Subsequently, comprehensive techno-economic modeling and analyses must be accomplished by accounting for capital and operation and maintenance costs, land costs, installation labor costs, etc. The financial feasibility of all hybrid configurations by estimating their Levelized Cost of Electricity (LCOE) will be assessed. Furthermore, the environmental modeling should be conducted calculating CO, CO2, NOx, and SOx emissions and the total costs of their pollution damages. It may require implanting carbon capture technologies to mitigate the probable environmental impacts of some hydrogen generation facilities. Finally, detailed and comprehensive results should be provided for future studies.
Engineering Equation Solver (EES), MATLAB, and Aspen Plus can be used for mathematical modelings and simulations in both WP2 and WP3.
WP4 (Sustainability Analysis)[edit | edit source]
Analytical tools of the sustainability assessment can help the researchers to model the hybrid systems in a quantitative or qualitative way aiming at providing technical information for a better decision. Different sustainability analyses based on physical metrics, including but not limited to Life Cycle Assessment (LCA) or carbon footprint, Environmental Risk Assessment, and Ecological Footprint, should be carried out to provide us the sustainability level of the hybrid multi-generation systems.
WP5 (Multi-objective Optimization)[edit | edit source]
In order to select the best hybrid configuration for the under-study circumstances, the optimization problem should be defined for all of the proposals considering various criteria (energy, economic, and environmental) as the objective functions of our problem. To formulate the optimization problem, the MATLAB optimization toolbox, which is based on GAMS, can be employed. Accordingly, the most optimal solar-based hybrid multi-generation system(s) from different perspectives would be chosen to apply the last step of modeling on it (them).
WP6 (Predictive Control Modeling of Smart Hybrid Systems)[edit | edit source]
Transient modeling of the optimal solar-based hybrid cycle(s) could be carried out using TRANSYS software aiming at the depiction of realistic performance of that (these) hybrid system(s). In particular, the role of a solar-based multi-generation system as the core of an industrial/residential cluster will be assessed. The off-grid potentials of the proposed systems will be studied, and for on-grid community-scaled proposals, further studies should be done on the power transmission challenges. Ultimately, the predictive control modeling of the chosen hybrid system(s) can be implemented employing Machine Learning and Deep Learning strategies via Python or MATLAB softwares in order to provide a/the smart optimized solar-based multi-generation system(s)
Work packs.
WP1 | WP2 | WP3 | WP4 | WP5 | WP6 |
---|---|---|---|---|---|
Detailed Literature Review | Preliminary Modeling Based on the Experimental Investigations | Advanced Economic and Environmental Modeling | Sustainability Analysis | Multi-objective Optimization | Predictive Control Modeling of Smart Hybrid Systems |
Benefits[edit | edit source]
When such a comprehensive project is completed, copious useful benefits would be provided for scientists, decision makers, politicians, and subsequently for world people in the long term:
First of all, several PhD and MSc students can get involved in this project, defining their theses based on the objectives of its different work packs. Furthermore, various universities, research institutes, and industrial centers can contribute to this project and gain great scientific, financial, and competitive benefits by fulfilling their commitments.
Although governments should invest in promoting some novel technologies, the profitable outcomes of this project will satisfy their economic goals. Undoubtedly, exploiting freely available natural resources by establishing sustainable hybrid systems will lead to significantly lower operational costs and greenhouse gas emissions attributed to conventional fossil fuel power plants.
In today's world, the values of diversified products, including electricity, heating/cooling, and HVAC facilities, freshwater, hydrogen, and other fuels, which are produced employing sustainable technologies, can not be evaluated by other things.
Last but not least, the proposed research is so important since its achievements will pave the governments' way toward having a cleaner world. Thus, in the long term, world people will benefit from a clean, safe, and reliable world, in which they can afford their energy costs, as well.
References[edit | edit source]
- ↑ Zepf V. The dependency of renewable energy technologies on critical resources. Elsevier Inc.; 2020. https://doi.org/10.1016/b978-0-12-819534-5.00004-0
- ↑ 2.0 2.1 2.2 Moriarty P, Honnery D. 6 - Global renewable energy resources and use in 2050. Elsevier Inc.; 2019. https://doi.org/10.1016/B978-0-12-814104-5.00006-5
- ↑ 3.0 3.1 3.2 Solar Energy n.d. https://www.appropedia.org/Solar_energy (accessed October 17, 2021)
- ↑ 4.0 4.1 Tiwari GN, Tiwari A, Shyam. Solar Water-Heating Systems Á Natural circulation Á Forced circulation Á Heat. 2016
- ↑ 5.0 5.1 5.2 The Challenges of Solar Energy n.d. https://www.fuergy.com/blog/the-challenges-of-solar-energy (accessed October 17, 2021)
- ↑ de Falco M, Mastrandrea N. An Analytical Model for Optimizing the Combination of Energy Sources in a Single Power Transmission Network. J Renew Energy 2014;2014:1–10. https://doi.org/10.1155/2014/143736
- ↑ Wikimdeia Commons n.d. https://commons.wikimedia.org/wiki/File:Iceland_Geothermal_facility.jpg (accessed October 18, 2021).
- ↑ Geothermal Energy in Iceland n.d. http://large.stanford.edu/courses/2018/ph240/billman1/ (accessed October 17, 2021)
- ↑ Ghiasirad H, Asgari N, Khoshbakhti Saray R, Mirmasoumi S. Thermoeconomic assessment of a geothermal based combined cooling, heating, and power system, integrated with a humidification-dehumidification desalination unit and an absorption heat transformer. Energy Convers Manag 2021;235:113969. https://doi.org/https://doi.org/10.1016/j.enconman.2021.113969
- ↑ Cleary K, Palmer K. Renewables 101: Integrating Renewable Energy Resources into the Grid How Is Renewable Energy Integrated into the Grid? 2020
- ↑ Recharge n.d. https://www.rechargenews.com/transition/vanadium-flow-battery-giant-to-go-head-to-head-with-li-ion-kings/2-1-789769 (accessed October 18, 2021)
- ↑ Mahlia TMI, Saktisahdan TJ, Jannifar A, Hasan MH, Matseelar HSC. A review of available methods and development on energy storage; technology update. Renew Sustain Energy Rev 2014;33:532–45. https://doi.org/https://doi.org/10.1016/j.rser.2014.01.068
- ↑ Haas J, Cebulla F, Cao K, Nowak W, Palma-Behnke R, Rahmann C, et al. Challenges and trends of energy storage expansion planning for flexibility provision in low-carbon power systems – a review. Renew Sustain Energy Rev 2017;80:603–19. https://doi.org/https://doi.org/10.1016/j.rser.2017.05.201
- ↑ Energy storage n.d. https://www.appropedia.org/Energy_storage
- ↑ Fathima AH, Palanisamy K. Renewable systems and energy storages for hybrid systems. Elsevier Ltd; 2018. https://doi.org/10.1016/B978-0-08-102493-5.00008-X
- ↑ 16.0 16.1 Figaj R, Vanoli L. Hybrid and novel solar hydrogen systems. Sol Hydrog Prod Process Syst Technol 2019:487–510. https://doi.org/10.1016/B978-0-12-814853-2.00013-8
- ↑ Calise F, Cappiello FL, Vicidomini M. Chapter 9 - Applications of solar PV systems in hydrogen production. In: Gorjian S, Shukla A, editors. Photovolt. Sol. Energy Convers., Academic Press; 2020, p. 275–312. https://doi.org/https://doi.org/10.1016/B978-0-12-819610-6.00009-0
- ↑ Soltani M, Dehghani-Sanij A, Sayadnia A, Kashkooli FM, Gharali K, Mahbaz S, et al. Investigation of Airflow Patterns in a New Design of Wind Tower with a Wetted Surface. Energies 2018;11. https://doi.org/10.3390/en11051100
- ↑ Razmi A, Soltani M, Aghanajafi C, Torabi M. Thermodynamic and economic investigation of a novel integration of the absorption-recompression refrigeration system with compressed air energy storage (CAES). Energy Convers Manag 2019;187:262–73. https://doi.org/https://doi.org/10.1016/j.enconman.2019.03.010
- ↑ Mohammadi K, Saghafifar M, Ellingwood K, Powell K. Hybrid concentrated solar power (CSP)-desalination systems: A review. Desalination 2019;468:114083. https://doi.org/https://doi.org/10.1016/j.desal.2019.114083
- ↑ Chitgar N, Emadi MA, Chitsaz A, Rosen MA. Investigation of a novel multi-generation system driven by a SOFC for electricity and fresh water production. Energy Convers Manag 2019;196:296–310. https://doi.org/https://doi.org/10.1016/j.enconman.2019.06.006
- ↑ Iaquaniello G, Salladini A, Mari A, Mabrouk AA, Fath HES. Concentrating solar power (CSP) system integrated with MED–RO hybrid desalination. Desalination 2014;336:121–8. https://doi.org/https://doi.org/10.1016/j.desal.2013.12.030
- ↑ Ramjeawon T. Introduction to Sustainability for Engineers. n.d
Contact details[edit | edit source]
Please contact me for further information: nima.asgari1394[at]gmail.com