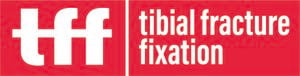
This module allows traditional bone setters, pre-hospital providers, clinical officers, nurses, nurse practitioners, and medical officers to become confident and competent in performing point-of-care ultrasound diagnostic imaging to rule out the presence of a pediatric distal forearm fracture and distinguish between buckle (torus) fractures and cortical break fractures to make appropriate referrals as part of the management of closed pediatric (< 16 years of age) distal forearm fractures in regions without access to X-ray imaging and orthopedic specialist coverage.[1][2][3][4][5][6][7][8][9]
Overview[edit | edit source]
Global Impact[edit | edit source]
A systematic review of 204 countries estimated there were 455 million prevalent cases of acute or long-term symptoms of a fracture in 2019.[10] The 2019 global incidence of fractures of the radius, ulna, or both was 30.7 million cases resulting in an estimated 210,000 years lived with disability (YLD). The distal forearm fracture is the most common childhood fracture (40%) and is routinely treated in every outpatient clinic.[11] Distal forearm fractures comprise 74% of all pediatric fractures in the upper extremity and the incidence of pediatric distal forearm fractures has been increasing despite global efforts to promote childhood safety.[12][13][14][15][16][17][18]
Access to high-quality orthopedic care in low to middle income countries (LMICs) is limited by a lack of providers, resources, and training programs.[19] The global standard is a ratio of one orthopedic surgeon to 200,000 people.[20] In 2008, Uganda reported a ratio of 1 orthopedic surgeon for every 1.3 million people.[21] In 2020, Nigeria had an estimated population of over 206 million people.[22] According to the Nigerian Orthopaedic Association, Nigeria's ratio is 1 orthopedic surgeon to approximately 500,000 people.[20] An estimated 75% of Nigerian orthopedic surgeons are located in 4 major cities which leaves other urban areas and rural regions without coverage.[23]
The national shortages of orthopedic surgeons in LIMCs leaves patients vulnerable to traditional bone setters whose unsafe practices result in worse outcomes compared to no treatment and commonly lead to malunion, limb shortening, gangrene, limb loss, and death.[24][25][26][27][28] A 2007 study of Nigerian healthcare institutions found unacceptably high rates of amputation (57% to 77.8%) and mortality (11.1% to 26.7%) secondary to bone setter's gangrene.[24] A 2004 study on 82 Nigerian patients (median age of 27 years) found that the leading cause for limb amputation (32%) was gangrene resulting from treatment of extremity injuries by traditional bone setters.[25] Since medical officers do not receive adequate exposure to orthopedic surgery during their undergraduate medical education, they often refer fracture patients to traditional bone setters in regions without orthopedic specialist coverage.
To compound matters, an estimated two-thirds of the world’s population (3.5–4.7 billion people) lacks access to any diagnostic imaging.[11][29] Handheld ultrasound devices can provide diagnostic imaging at the point-of-care for almost any musculoskeletal injury in resource-constrained settings, particularly where trauma is a primary cause of morbidity and mortality.[11] A high-frequency linear transducer is used for fracture sonography, ideally with at least 10 MHz frequency for better resolution for subtle fractures.[8][30] Nearly almost any commercially available portable ultrasound devices could be used in the musculoskeletal examination of an injured patient.
Ultrasound has been shown to be most effective for the diagnosis of long bone fractures and is capable of detecting fractures as small as 1 mm.[31] Ultrasound can detect even subtle fractures that may not be seen on x-ray imaging.[31] Children < 12 years of age generally sustain buckle (torus) fractures of the distal radius; and children 12 years and older have more physeal fractures due to the strengthening of bone in comparison to the weaker cartilaginous physis.[32] Ultrasound imaging should be guided by the clinical assessment of the child. The pain and/or swelling should be localised to the distal forearm and there should be no visible deformity. Carpal or mid/proximal forearm pain should prompt alternative imaging.[8]
While fracture ultrasonography can be performed across all patient populations, it is particularly beneficial for children.[11] Pediatric fracture lesions always cause alterations to the bone's surface which can be accurately detected on ultrasound.[11][33] Since up to 80% of X-rays obtained in children are negative for fractures and joint fractures are uncommon in patients aged 12 or younger with a low-risk injury mechanism, sonography offers an alternative, reliable method to diagnose fractures, and minimize the number of X-rays and exposure to ionizing radiation in radiation-sensitive children.[11][34][33][35] Sonography can be performed at the bedside, and thereby, eliminates the need to transport a child for imaging.[11] Ultrasound imaging is very well tolerated in children when copious amounts of ultrasound gel is used with the probe, the sonographer's scanning hand rests on an non-injured area, minimal pressure is applied to the injured region, and the child is permitted to remain in a comfortable position, typically next to or on a parent's lap.
Fracture sonography cannot be used universally, but only when there is high quality evidence demonstrating its effectiveness and safety.[11] Sonographic diagnosis must be proven independently for each anatomic location and each fracture type. Fracture sonography can replace X-rays for certain indications, but overall, sonography complements radiographs and does not replace them. If there is any doubt about a sonographic finding, an X-ray image should be obtained. The practitioner must still have access to X-ray imaging on-site or via referral at all times.
An evidence-based clinical guide shows that ultrasound diagnosis can or could replace X-rays for specific indications which are outlined in Table 1 below:[11]
Fracture Category[10] | Global Incidence in 2019[10] | Years Lived with Disability (YLD) in 2019 (95% Uncertainty Interval)[10] | Ultrasound Application[11] | Evidence Level[11][36] | Special Notes[11] |
---|---|---|---|---|---|
Fracture of skull | 7.59 million cases | 213,000 YLD (144,000 –308,000 YLD) | X-ray-free diagnosis of skullcap (dome) fractures in patients ages 0-18 years | Ia | Several studies showed a sensitivity of 88% and a specificity of 97% for sonography of skull cap fractures.[11] |
Fracture of sternum or fracture of one or more ribs, or both | 4.11 million cases | 191,000 YLD (128,000 –272,000 YLD) | X-ray-free diagnosis of rib and sternal fractures in patients of any age | Ia | "In general, sonography is superior to conventional X-ray diagnostics for mild and moderate trauma and can therefore generally be used as a primary diagnostic tool."[11] |
Fracture of clavicle, scapula, or humerus | 19.3 million cases | 247,000 YLD (151,000 –386,000 YLD) | X-ray-free diagnosis of clavicle fractures in newborns | IIa | "Compared to an X-ray, ultrasonography can accurately detect clavicular fractures in newborns, so that the connection between clinical and sonographic diagnostics is sufficient for diagnosis and documentation."[11] |
Fracture of radius or ulna, or both | 30.7 million cases | 210,000 YLD (131,000 –325,000 YLD) | X-ray-free exclusion of a fracture close to the elbow by diagnosis of the intra-articular effusion in patients ages 0-12 years | IIa | Use Elbow SAFE Algorithm.[11] |
Fracture of hand, wrist, or other distal part of hand | 19.0 million cases | 301,000 YLD (160,000 –522,000 YLD) | X-ray-free diagnosis and therapy of distal wrist fractures in patients ages 0-12 years | Ia | Use Wrist SAFE Algorithm.[11] Compared to X-ray imaging, sonography for distal wrist fractures has a sensitivity of 96%, specificity of 10%, a positive predictive value of 1, and negative predictive value of 0.88.[33][37] |
Determination of the axis deviation in subcapital metacarpal 5 fractures (boxer fracture) in patients of any age | V | "The indication for sonographic assessment is given in all cases with an unclear surgical indication. Due to the problems of X-ray imaging, the findings should be confirmed sonographically with every decision regarding conservative therapy."[11] | |||
Fracture of hip | 14.2 million cases | 2.94 million YLD (2.03–3.96 million YLD) | X-ray-free diagnosis and therapy for injuries to the lower extremity in patients ages 0-8 years | not provided |
|
Fracture of femur, other than femoral neck | 14.6 million cases | 1.85 million YLD (1.23–2.64 million YLD) | X-ray-free diagnosis and control of distal femoral torus fractures in patients ages 0-12 years | IV | "The indication for sonographic assessment is possible in children up to the age of 10 and in slim patients up to the age of 12. The diagnosis is made using classic sonographic fracture signs. If the course is uncomplicated, an X-ray diagnosis is not mandatory."[11] |
Fracture of patella, tibia or fibula, or ankle | 32.7 million cases | 15.5 million YLD (10.2–22.6 million YLD) | Additional diagnostics in the event of clinical suspicion of a proximal tibia torus fracture in patients up to the age of 12 | V | "Torus fractures are often only discreet in the X-ray image. There is usually a minimal bulge in the AP picture, while the side picture is completely unremarkable. [F]racture sonography can quickly provide a reliable diagnosis and make the bulge clearly visible. Since there are no randomized studies on this indication, the accompanying X-ray diagnosis is still mandatory. However, it is to be expected that ultrasound can also be used for exclusion diagnosis at this point in the future and will also be used as sole imaging in the case of torus fractures."[11] |
Fracture of foot bones except ankle | 10.7 million cases | 480,000 YLD (291,000 – 750,000 YLD) | Position control, and detection/exclusion of a dislocation in a conservatively treated, radiologically proven MFK 5 base fracture in patients of any age | IIa | "Since no blinded studies have yet been published on this indication, exact information on the safety of the method compared to the X-ray display is not available. However, the good visualization of the structures and the simple technology make it very likely that the display will achieve a comparable or better quality than the conventional X-ray image."[11] |
Total: | 153 million cases | 22.0 million YLD (14.5 - 31.8 million YLD) |
The sonographic diagnosis of pediatric distal forearm fractures is the ideal entry-level indication for novices to fracture sonography because of the frequent occurrence of this injury, the rapid (~1 minute) and painless application of this examination, the bone contour and thin soft tissue envelope enables reliable sonographic diagnosis, and the distal wrist can tolerate axis deviations of up to 40° until the patient reaches 12 years of age.[11] A 2022 Australian study demonstrated that nurse practitioners with no prior sonographic experience achieved a mean diagnostic accuracy of 90% in the sonographic examination of pediatric distal forearm injuries after a 2 hour training course, practical training on each other's upper extremities and simulation models made from animal bones, 3 proctored ultrasound exams on patients, and 15 independent scans on patients.[6]
The high level evidence showing sonography can replace X-rays for the diagnosis of pediatric distal forearm fractures makes this indication suitable for traditional bone setters, prehospital providers, clinical officers, nurses, nurse practitioners, and medical officers who work at community facilities and primary health centers where X-ray equipment is not available on-site but can be accessed via referral.[1][2][3][4][5][6][7][11][38] As more evidence accumulates for using sonography as the sole imaging modality for other indications (see table above), additional ultrasound skills training modules can be developed for these traditional and orthodox practitioners.[1][2][3][4][5][6][7][11][35]
The management of non-displaced fractures is one of the 44 essential surgical procedures identified by the World Bank.[39] This module uses locally reproducible, high-fidelity, 3D printed simulators with targeted feedback to train healthcare providers to perform point-of-care fracture sonography and apply soft, simple bandaging of pediatric, closed, non-displaced buckle fractures of the distal forearm, and make appropriate referrals as part of the management of pediatric distal forearm fractures to prevent disability and limb loss.[40] This module teaches psychomotor skills that are transferable to the performance of other limb-saving procedures that require point-of-care ultrasound diagnosis. These skills can be used to prevent needless suffering, disability, and deaths for the estimated 153 million patients who sustain skull, sternal, rib and appendicular skeletal fractures globally every year.[10]
Disability Adjusted Life Years (DALY) Burden in LMICs[edit | edit source]
The estimated 2019 DALY burden of the procedure gap for the diagnosis and management of pediatric distal forearm contusions and fractures in LMICs is 2.00 million DALY with a 95% uncertainty interval of 1.61 - 2.59 million DALY. Our DALY estimates are based on data published in the peer-reviewed literature by authors who are not members of the Tibial Fracture Fixation team. Estimates are rounded to three significant figures for counts and one decimal place for percent values. We have outlined our methodology for estimating the DALY burden in LMICs below:
A 2021 Swedish Fracture Register study on 295,713 skeletal fractures sustained in adult patients (>= 16 years of age) from 2012 to 2018 found 50,610 cases of distal radius fractures.[41] Therefore, fractures of the distal radius account for 17.1% of adult skeletal fractures (50,610/295,713 x 100%) in this national registry. A 2021 systematic review of 204 countries estimated there were 156 million cases of new skeletal fractures with a 95% uncertainty interval of 124 - 196 million cases in 2019.[10] Based on the 2021 Swedish Fracture Register study, we estimate there were 26.7 million adult cases of new distal radius fractures (156 million x 17.1%) with a 95% uncertainty interval of 21.2 - 33.5 million cases worldwide in 2019.[41]
The 2019 global incidence of fractures of the radius or ulna or both was 30.7 million cases with a 95% uncertainty interval of 24.5 - 38.6 million cases.[10] Therefore, we estimate the total number of pediatric distal forearm fractures worldwide in 2019 was 4.00 million cases (30.7-26.7 million cases) with a 95% uncertainty interval of 3.30 - 5.10 million cases. A 2022 Turkish study on 3,261 pediatric fracture patients (mean age = 9.8 years) found that distal radius and ulna fractures were the most common fracture type (23%) and the overall mortality rate of pediatric fractures is 0.1%.[42] Assuming Japanese female life expectancy is 88 years, we estimated the years of life lost (YLL) for the procedure gap for the diagnosis and management of pediatric distal forearm fractures is 313,000 YLL with a 95% uncertainty interval of 258,000 YLL - 399,000 YLL.[43]
The 2019 global prevalence of fractures of the radius or ulna or both resulted in an estimated 210,000 years lived with disability (YLD) with a 95% uncertainty interval of 131,000 - 325,000 YLD.[10] We estimated the YLD (210,000 years x 4.00 million cases/30.7 million cases) to be 27,362 YLD with a 95% uncertainty interval of 17,068 YLD to 42,345 YLD.
We estimated the 2019 DALY global burden of the procedure gap for the diagnosis and management of pediatric distal forearm fractures is 340,362 DALY (YLL + YLD) with an 95% uncertainty interval of 275,068 DALY to 441,345 DALY. The World Bank estimates the total population in LMICs is 6.57 billion which is 83.8% of the world's total population of 7.84 billion.[44][45] Thus, we estimated 2019 DALY burden of the procedure gap for the diagnosis and management of pediatric distal forearm fractures in LMICs is 285,223 DALY (340,362 DALY x 83.8%) with a 95% uncertainty interval of 230,507 DALY to 369,847 DALY.
A 2019 national U.S. study on emergency departments found that the ratio of wrist contusions to wrist fractures in patients under the age of 18 was 6:1 (42,391 cases to 7,047 cases).[46] Assuming that the same DALY burden for pediatric distal forearm fractures applies to pediatric distal forearm contusions in LMICs, then the estimated 2019 DALY burden of the procedure gap for the diagnosis and management of pediatric distal forearm contusions and fractures in LMICs is 2.00 million DALY ([285,223 DALY x 6] + 285,223 DALY) with a 95% uncertainty interval of 1.61 million DALY to 2.59 million DALY.
This module teaches psychomotor skills that are transferable to the performance of ultrasound diagnosis of other fracture sites in children and adults listed in Table 1.[11] The estimated YLD portion of the 2019 DALY burden of the procedure gap for evidence-based ultrasound diagnosis of skull, sternal, rib and appendicular skeletal fractures in LMICs is 16.8 million YLD with a 95% uncertainty interval of 12.2 - 26.6 million YLD.[10][11] Estimates are rounded to three significant figures for counts and one decimal place for percent values. We have outlined our methodology for estimating the YLD portion of the DALY burden in LMICs below:
We estimated the YLD portion of the 2019 DALY burden of the procedure gap for the ultrasound diagnosis of skull, sternal, rib and appendicular skeletal fractures globally using previously published estimates of 22.0 million YLD with a 95% uncertainty interval of 14.5 - 31.8 million YLD.[10] The World Bank estimates the total population in LMICs is 6.57 billion which is 83.8% of the world's total population of 7.84 billion.[44][45] We estimated the YLD portion of the 2019 DALY burden of the procedure gap for the ultrasound diagnosis of skull, sternal, rib and appendicular skeletal fractures in LMICs by multiplying the YLD value x 83.8%.
Open-Source 3D Printing Technologies for Ultrasound Simulation Training[edit | edit source]
Fracture sonography skills will be acquired by the learner through knowledge review of online, open-access ultrasound images, practical training on learner's upper extremities to distinguish soft tissue features from bone, unblinded training with a range of fracture subtype simulators made from 3D printed bone models encased in clear gelatin and blinded training with a range of fracture subtype simulators made from 3D printed bone models encased in opaque gelatin.[6][7][9] Unlike animal bone models, our cruelty-free, hygienic, and reusable 3D printed bone models display both the radius and ulna, accurately represent bone length and diameter, external contour and cross-sectional shape, simulate the physis (growth plate), a normal feature which the learner must be able to distinguish from a fracture, and can consistently reproduce a wide range of fractures, including a proximal forearm fracture and a commonly missed greenstick fracture type.[6][7][9]
Open-source 3D printing technology supports the local, digital fabrication of the highest fidelity bone simulation models at the lowest cost.[47] The Pediatric Forearm Simulators are composed of reusable, 3D printed fracture models that mimic bone sonographically, are housed within a low-cost gelatin-based solution that simulates soft tissue, and are created by using reusable 3D printed open-face, negative moulds.[2][3][4][48][7][9][49][50]
Open-source, open filament and user-friendly desktop 3D printers are currently in use at small to medium enterprises, Makerspaces, start-up incubators, universities, and hospitals worldwide.[51][52][53][54][55][56][57][58][59][60][61][62][63] Our 3D printed bone simulation models are designed to reduce simulator costs, simplify the simulator build, and minimize simulator assembly time for the learner. This module provides an open source library of downloadable, 3D printed age and gender-specific bone simulation models and soft tissue moulds which accurately represent bone length and diameter, external contour and cross-sectional shape, cortical hardness, physeal width, common fracture subtypes, and forearm and wrist circumference values at ultrasound scanning sites for pediatric distal forearm fractures.[18][64][65][66][67][68][69][70][71][72]
All of the module's 3D printed models can be locally reproduced on open source, open filament, user-friendly, fused deposition modelling, single extruder desktop 3D printers that print polylactic acid (PLA), a low-cost, biorenewable, and biodegradable plastic.[73][74][75][76][77] According to one filament manufacturer, 3D printed PLA at 100% infill has a Shore Hardness D value of 83D and 84D while independently measured Shore Hardness D values of 3D printed PLA samples range from 80D to 88D (n=12).[66][67][68] These Shore Hardness D values of 3D printed PLA are within the 3-sigma range for the Shore Hardness D measurements of 86.7D + 1.91D (ave. ± s.d., n=1815) for human cortical bone.[69] To maximize the likelihood that these 3D printed models provide similar tactile and sonographic feedback as human bone and do not foster the development of anti-skills, the bone simulation models are made from PLA, a plastic filament with a hardness level similar to cortical bone, and that mimics bone sonographically.[48][66][67][68][69]
One shortcoming of our Pediatric Forearm Simulators in teaching the performance of fracture sonography skills is that our 3D printed bone models require radiopaque 3D printed male parts to connect the metaphysis across the radiolucent physis to the epiphysis of each forearm bone. This module informs the learner that the 3D printed male parts that connect the epiphysis to the metaphysis will be visible during simulation skills training but would not be visible when performing ultrasound scans on live pediatric patients. Another shortcoming of our prototype Pediatric Forearm Simulators is that the gelatin-based soft tissue simulation layer does not sonographically display the soft tissue features of pediatric distal forearm fractures, such as the pronator quadratus hematoma (PQH) sign.[78] Instead, this module instructs learners to practise on each other to identify and compare a normal pronator quadratus muscle in both upper extremities and provides the learner with online, open-access ultrasound images to learn to identify the sonographic features of the PQH sign.
The user's learnings on high fidelity 3D printed bone simulation models will translate into the clinical performance of the 4 training skills objectives for the Ultrasound Diagnosis of Pediatric Distal Forearm Fractures:
- Identify the primary sonographic features of no fracture, a buckle (torus) fractures and a cortical break fracture of the pediatric distal forearm;
- Describe the secondary sonographic features of a cortical break fracture of the pediatric distal forearm;
- Perform the 6 view method for ultrasonographic diagnosis of pediatric distal forearm fractures; and
- Practise conducting a bilateral ultrasound scan to diagnose a pronator quadratus hematoma.
Pre-Learning Clinical Confidence Assessment[edit | edit source]
Before the learner starts this module:
- Go to this link.
- Download, print out, and complete the pre-learning clinical confidence assessment for this training module.
- Photograph the completed assessment on your cellphone as a backup and file the assessment in your training records.
Phase 1: Knowledge Review[edit | edit source]
It is highly recommended that the learner be familiar with this content before proceeding to the skill pages.
Phase 2: Simulator Build[edit | edit source]
Instructions for 3D Printing Organizations[edit | edit source]
Please pay attention to and follow all the instructions closely to ensure the models are printed properly.
Simulator Assembly[edit | edit source]
Portable Ultrasound Devices[edit | edit source]
- Butterfly iQ Ultrasound
- GE Vscan Air
- Kosmos Lexsa Ultrasound Probe
- Sonosite M-Turbo or Sonosite iViz
- Philips Lumify
Phase 3: Skills Practice[edit | edit source]
Phase 4: Self-Assessment[edit | edit source]
The module’s self-assessment framework includes a checklist and labelled image review so the user can self-generate targeted feedback which enables the user to: ensure they are practicing the appropriate skills; modify their performance to improve competence; and determine when they have practiced to a sufficient level of mastery to perform the procedure in a patient.
Training Module Certificate of Completion[edit | edit source]
Once the self-assessment framework has been completed:
- Go to this link.
- Click on "Get your certificate" button under the "Menu" section in the upper right corner of the module page.
- Type in your name, download and print out a certificate of completion for this training module.
- Photograph your certificate on your cellphone as a backup and file the printed certificate in your training records.
Post-Learning Clinical Confidence Assessment[edit | edit source]
After the learner completes this module:
- Go to this link.
- Download, print out, and complete the post-learning clinical confidence assessment for this training module.
- Photograph the completed assessment on your cellphone as a backup and file the assessment in your training records.
If the learner does not feel confident in performing this procedure in real clinical scenarios, the learner can repeat this module and practice the skills training as many times as they feel necessary to gain the confidence to perform this procedure on patients.
Supplemental Learning Topics[edit | edit source]
(Optional) After completion of the module, the learner may wish to learn more about 3D printing technology:
Additional Module Information[edit | edit source]
(Optional) After completion of the module, the learner may wish to learn more about this module:
Follow-on[edit | edit source]
(Optional) After completion and practice to competency, the learner may wish to continue study with these courses:
Acknowledgements[edit | edit source]
This work is funded by a grant from the Intuitive Foundation. Any research, findings, conclusions, or recommendations expressed in this work are those of the author(s), and not of the Intuitive Foundation.
References[edit | edit source]
- ↑ 1.0 1.1 1.2 Onyemaechi NO, Itanyi IU, Ossai PO, Ezeanolue EE. Can traditional bonesetters become trained technicians? Feasibility study among a cohort of Nigerian traditional bonesetters. Hum Resour Health. 2020 Mar 20;18(1):24. doi: 10.1186/s12960-020-00468-w. PMID: 32197617; PMCID: PMC7085192.
- ↑ 2.0 2.1 2.2 2.3 Heiner JD, McArthur TJ. The ultrasound identification of simulated long bone fractures by prehospital providers. Wilderness Environ Med. 2010 Jun;21(2):137-40. doi: 10.1016/j.wem.2009.12.028. Epub 2009 Dec 22. PMID: 20591377.
- ↑ 3.0 3.1 3.2 3.3 Heiner JD, Baker BL, McArthur TJ. The ultrasound detection of simulated long bone fractures by U.S. Army Special Forces Medics. J Spec Oper Med. 2010 Spring;10(2):7-10. PMID: 20936597.
- ↑ 4.0 4.1 4.2 4.3 Heiner JD, Proffitt AM, McArthur TJ. The ability of emergency nurses to detect simulated long bone fractures with portable ultrasound. Int Emerg Nurs. 2011 Jul;19(3):120-4. doi: 10.1016/j.ienj.2010.08.004. Epub 2010 Sep 25. PMID: 21665155.
- ↑ 5.0 5.1 5.2 Snelling PJ, Jones P, Keijzers G, Bade D, Herd DW, Ware RS. Nurse practitioner administered point-of-care ultrasound compared with X-ray for children with clinically non-angulated distal forearm fractures in the ED: a diagnostic study. Emerg Med J. 2021 Feb;38(2):139-145. doi: 10.1136/emermed-2020-209689. Epub 2020 Sep 8. PMID: 32900856.
- ↑ 6.0 6.1 6.2 6.3 6.4 6.5 Snelling PJ, Jones P, Moore M, Gimpel P, Rogers R, Liew K, Ware RS, Keijzers G. Describing the learning curve of novices for the diagnosis of paediatric distal forearm fractures using point-of-care ultrasound. Australas J Ultrasound Med. 2022 Mar 7;25(2):66-73. doi: 10.1002/ajum.12291. PMID: 35722050; PMCID: PMC9201201.
- ↑ 7.0 7.1 7.2 7.3 7.4 7.5 Heiner JD, McArthur TJ. A simulation model for the ultrasound diagnosis of long-bone fractures. Simul Healthc. 2009 Winter;4(4):228-31. doi: 10.1097/SIH.0b013e3181b1a8d0. PMID: 19915442.
- ↑ 8.0 8.1 8.2 Snelling PJ, Keijzers G, Byrnes J, Bade D, George S, Moore M, Jones P, Davison M, Roan R, Ware RS. Bedside Ultrasound Conducted in Kids with distal upper Limb fractures in the Emergency Department (BUCKLED): a protocol for an open-label non-inferiority diagnostic randomised controlled trial. Trials. 2021 Apr 14;22(1):282. doi: 10.1186/s13063-021-05239-z. PMID: 33853650; PMCID: PMC8048294.
- ↑ 9.0 9.1 9.2 9.3 Snelling PJ. A low-cost ultrasound model for simulation of paediatric distal forearm fractures. Australas J Ultrasound Med. 2018 Feb 25;21(2):70-74. doi: 10.1002/ajum.12083. PMID: 34760505; PMCID: PMC8409885.
- ↑ 10.0 10.1 10.2 10.3 10.4 10.5 10.6 10.7 10.8 10.9 Global, regional, and national burden of bone fractures in 204 countries and territories, 1990–2019: a systematic analysis from the Global Burden of Disease Study 2019. GBD 2019 Fracture Collaborators. Published: August 20, 2021. DOI:https://doi.org/10.1016/S2666-7568(21)00172-0.
- ↑ 11.00 11.01 11.02 11.03 11.04 11.05 11.06 11.07 11.08 11.09 11.10 11.11 11.12 11.13 11.14 11.15 11.16 11.17 11.18 11.19 11.20 11.21 11.22 11.23 11.24 11.25 11.26 Ackermann O. Fracture sonography: a comprehensive clinical guide. Cham (Switzerland): Springer Nature Switzerland AG; 2019, 2021. 163 p.
- ↑ Joeris A, Lutz N, Blumenthal A, Slongo T, Audigé L. The AO Pediatric Comprehensive Classification of Long Bone Fractures (PCCF). Acta Orthop. 2017;88(2):123–128.
- ↑ Sminkey L. World report on child injury prevention. Inj Prev. 2008;14(1):69.
- ↑ Kramhøft M, Bødtker S. Epidemiology of distal forearm fractures in Danish children. Acta Orthop Scand. 1988;59(5):557–559.
- ↑ Khosla S, Melton LJ, Dekutoski MB, Achenbach SJ, Oberg AL, Riggs BL. Incidence of childhood distal forearm fractures over 30 years: a population- based study. JAMA. 2003;290(11):1479–1485.
- ↑ Jónsson B, Bengnér U, Redlund- Johnell I, Johnell O. Forearm fractures in Malmö, Sweden. Changes in the incidence occurring during the 1950s, 1980s and 1990s. Acta Orthop Scand. 1999;70(2):129–132.
- ↑ Hedström EM, Svensson O, Bergström U, Michno P. Epidemiology of fractures in children and adolescents. Acta Orthop. 2010;81(1):148–153.
- ↑ 18.0 18.1 Korup LR, Larsen P, Nanthan KR, Arildsen M, Warming N, Sørensen S, Rahbek O, Elsoe R. Children's distal forearm fractures: a population-based epidemiology study of 4,316 fractures. Bone Jt Open. 2022 Jun;3(6):448-454. doi: 10.1302/2633-1462.36.BJO-2022-0040.R1. PMID: 35658607; PMCID: PMC9233428.
- ↑ Conway DJ, Coughlin R, Caldwell A, Shearer D. The Institute for Global Orthopedics and Traumatology: A Model for Academic Collaboration in Orthopedic Surgery. Front Public Health. 2017 Jun 30;5:146. doi: 10.3389/fpubh.2017.00146. PMID: 28713803; PMCID: PMC5491941.
- ↑ 20.0 20.1 Nigeria has 350 orthopaedic surgeons for 170 million citizens. (2016, November 26) Agency Report. Premium Times. Retrieved November 11, 2020, from https://www.premiumtimesng.com/news/more-news/216415-nigeria-350-orthopaedic-surgeons-170-million-citizens.html.
- ↑ Naddumba EK. Musculoskeletal trauma services in Uganda. Clin Orthop Relat Res. 2008 Oct;466(10):2317-22. doi: 10.1007/s11999-008-0369-2. Epub 2008 Jul 16. PMID: 18629599; PMCID: PMC2584282.
- ↑ Population, total - Nigeria [Internet]. Data. The World Bank; 2021 [cited 2021 Dec 10]. Available from: https://data.worldbank.org/indicator/SP.POP.TOTL?locations=NG.
- ↑ National Hospital records high patronage on knee, hip replacement surgeries. (2020, January 26) The Sun Nigeria. Retrieved November 11, 2020 from https://www.sunnewsonline.com/national-hospital-records-high-patronage-on-knee-hip-replacement-surgeries/.
- ↑ 24.0 24.1 Nwadiaro HC. Bone setter's gangrene. Nigerian Journal of Medicine Vol. 16 (1) 2007: pp. 8-10. DOI: https://doi.org/10.4314/njm.v16i1.37273.
- ↑ 25.0 25.1 Umaru RH, Gali BM, Ali N; (2004) Role of inappropriate traditional splintage in limb amputation in Maiduguri, Nigeria. Annals of African Medicine, 3(3):138-140. URL: http://www.bioline.org.br/request?am04034.
- ↑ Dada A, Giwa SO, Yinusa W, Ugbeye M, Gbadegesin S. Complications of treatment of musculoskeletal injuries by bone setters. West Afr J Med. 2009; 28(1):43–7.
- ↑ Mohamed Imad A, Hag EL, Osman Bakri M, Hag EL. Complications in fractures treated by traditional bonesetters in Khartoum, Sudan. Khartoum Med J. 2010;3(1):401–5.
- ↑ Onyemaechi NOC, Onwuasoigwe O, Nwankwo OE, Schuh A, Popoola SO. Complications of musculoskeletal injuries treated by traditional bonesetter in a developing country. Indian J Appl Res. 2014;4(3):313–6.
- ↑ World Health Organization. Baseline country survey on medical devices WHO global health observatory. Geneva: World Health Organization; 2010.
- ↑ Douma-den Hamer D, Blanker MH, Edens MA, Buijteweg LN, Boomsma MF, van Helden SH, et al. Ultrasound for distal forearm fracture: a systematic review and diagnostic meta-analysis. PLoS One. 2016;11(5):e0155659. doi: 10.1371/journal.pone.0155659.
- ↑ 31.0 31.1 Hübner U, Schlicht W, Outzen S, Barthel M, Halsband H. Ultrasound in the diagnosis of fractures in children. J Bone Joint Surg Br. 2000 Nov;82(8):1170-3. doi: 10.1302/0301-620x.82b8.10087. PMID: 11132281
- ↑ Kramhoft, M. S. Bodtker. Epidemiology of distal forearm fractures in Danish children. Acta Orthop Scand 1988;59(5);557-559.
- ↑ 33.0 33.1 33.2 https://en.wikipedia.org/wiki/Fracture_sonography
- ↑ Ingo Marzi: "Verletzungsformen" In: Kindertraumatologie Springer, Berlin/Heidelberg 2010. ISBN 978-3-642-00990-7, p 12.
- ↑ 35.0 35.1 Koetter, Paige BS; Gallo, Robert MD; Kasmire, Kathryn E. MD. Assessing the Necessity for the “Joint Above and Below” Radiography Approach for Lower-extremity Long Bone Fractures in Children. Pediatric Emergency Care: January 2022 - Volume 38 - Issue 1 - p e316-e320. doi: 10.1097/PEC.0000000000002274.
- ↑ http://www.cebm.net
- ↑ Ole Ackermann et al: Ist die Sonographie geeignet zur Primärdiagnostik kindlicher Vorderarmfrakturen? In: Deutsche Zeitschrift für Sportmedizin. 60, 2009, pp 355–358, ISSN 0344-5925.
- ↑ Ole Ackermann, Kolja Eckert: Sonographische Frakturdiagnostik im Kindesalter. In: Rupprecht (Hrsg) Pädiatrische Ultraschalldiagnostik. Ecomed-Verlag, Landsberg. 31. Ergänzungslieferung. 2014 ISBN 978-3-609-71602-2
- ↑ Debas, H. T., P. Donkor, A. Gawande, D. T. Jamison, M. E. Kruk, and C. N. Mock, editors. 2015. Essential Surgery. Disease Control Priorities, third edition, volume 1. Washington, DC: World Bank. doi:10.1596/978-1-4648 -0346-8. License: Creative Commons Attribution CC BY 3.0 IGO
- ↑ Perry DC, Achten J, Knight R, Appelbe D, Dutton SJ, Dritsaki M, Mason JM, Roland DT, Messahel S, Widnall J, Costa ML; FORCE Collaborators in collaboration with PERUKI. Immobilisation of torus fractures of the wrist in children (FORCE): a randomised controlled equivalence trial in the UK. Lancet. 2022 Jul 2;400(10345):39-47. doi: 10.1016/S0140-6736(22)01015-7. Erratum in: Lancet. 2022 Jul 23;400(10348):272. Erratum in: Lancet. 2022 Oct 1;400(10358):1102. PMID: 35780790.
- ↑ 41.0 41.1 Camilla Bergh, Michael Möller, Jan Ekelund & Helena Brisby (2021) 30-day and 1-year mortality after skeletal fractures: a register study of 295,713 fractures at different locations, Acta Orthopaedica, 92:6, 739-745, DOI: 10.1080/17453674.2021.1959003.
- ↑ Bilge O, Kekeç AF, Atılgan N, Yaka H, Dundar ZD, Karagüven D, et al. The initial analysis of pediatric fractures according to the AO/OTA fracture classification and mechanisms of injuries. Ulus Travma Acil Cerrahi Derg 2022;28:1500-1507.
- ↑ https://data.worldbank.org/indicator/SP.DYN.LE00.FE.IN?locations=JP
- ↑ 44.0 44.1 https://data.worldbank.org/indicator/SP.POP.TOTL
- ↑ 45.0 45.1 https://data.worldbank.org/country/XO
- ↑ Wenzinger E, Rivera-Barrios A, Gonzalez G, Herrera F. Trends in Upper Extremity Injuries Presenting to US Emergency Departments. Hand (N Y). 2019 May;14(3):408-412. doi: 10.1177/1558944717735943. Epub 2017 Nov 9. PMID: 29121783; PMCID: PMC6535948.
- ↑ Tibial Fracture Fixation/Innovative Design#Lower Cost
- ↑ 48.0 48.1 Beaulieu A, Linden Az, Phillips J, Arroyo LG, Koenig J, Monteith G (2019) Various 3D printed materials mimic bone ultrasonographically: 3D printed models of the equine cervical articular process joints as a simulator for ultrasound guided intra-articular injections. PLoS ONE 14(8): e0220332. https://doi.org/10.1371/journal.pone.0220332.
- ↑ Bude RO, Adler RS. An easily made, low-cost, tissue-like ultrasound phantom material [Internet]. Journal of clinical ultrasound: JCU. U.S. National Library of Medicine; 1995 [cited 2021 Nov 12]. Available from: https://pubmed.ncbi.nlm.nih.gov/7797668/.
- ↑ Culjat MO, Goldenberg D, Tewari P, Singh RS. A review of tissue substitutes for ultrasound imaging. Ultrasound Med Biol. 2010 Jun;36(6):861-73. doi: 10.1016/j.ultrasmedbio.2010.02.012. PMID: 20510184.
- ↑ https://world.prusa3d.com/
- ↑ https://3dafrica.org/
- ↑ https://impacthub.net/about-us-regions-locations/
- ↑ AIGE Limited. 3D printers. [Internet]. 3D Printers | AIGE Limited. [cited 2021 July 29]. Available from: https://www.aige.info/3d-printers.
- ↑ MoTIV. Motiv tribe [Internet]. MoTIV. 2021 [cited 2021 July 29]. Available from: https://motiv.africa/.
- ↑ https://www.creality3dofficial.com/products/official-creality-ender-3-3d-printer?gclid=EAIaIQobChMIn76Xn4_A9wIVD4FaBR0klwSJEAAYAyAAEgIzRvD_BwE
- ↑ Prusa J. Open-Source 3D printers from Josef Prusa [Internet]. Prusa3D. 2019 [cited 2021 July 29]. Available from: https://www.prusa3d.com/.
- ↑ https://lulzbot.com/store/sidekick747?ref=null
- ↑ Ultimaker. Ultimaker 3D printers: Reliable and easy to use [Internet]. ultimaker.com. [cited 2021 July 29]. Available from: https://ultimaker.com/3d-printers.
- ↑ https://www.fablabs.io/labs/map
- ↑ https://wiki.hackerspaces.org/List_of_Hacker_Spaces
- ↑ https://makerspaces.make.co/
- ↑ Niatech. CoRSU Starts Field Testing 3D Printed Orthopaedic Devices For Children With Disabilities [Internet]. Canada: Niatech; June 13, 2016 [cited 2020 Nov 11]. Available from: https://niatech.org/corsu-starts-field-testing-3d-printed-orthopaedic-devices-for-children-with-disabilities/.
- ↑ Gindhart PS. Growth standards for the tibia and radius in children aged one month through eighteen years. Am J Phys Anthop 1973; 39(1): 41–8.
- ↑ https://3dprint.nih.gov/discover/3dpx-016807
- ↑ 66.0 66.1 66.2 Ultimaker. Ultimaker PLA Technical Data Sheet [Internet]. Ultimaker Support. [cited 2021 July 29]. Available from: https://support.ultimaker.com/hc/en-us/articles/360011962720-UltimakerPLA-TDS.
- ↑ 67.0 67.1 67.2 https://support.ultimaker.com/hc/en-us/articles/360011962720-Ultimaker-PLA-TDS
- ↑ 68.0 68.1 68.2 Vian, Wei Dai and Denton, Nancy L., "Hardness Comparison of Polymer Specimens Produced with Different Processes" (2018). ASEE IL-IN Section Conference. 3. https://docs.lib.purdue.edu/aseeil-insectionconference/2018/tech/3.
- ↑ 69.0 69.1 69.2 Society For Biomaterials 30th Annual Meeting Transactions, page 332. Femoral Cortical Wall Thickness And Hardness Evaluation. K. Calvert, L.A. Kirkpatrick, D.M. Blakemore, T.S. Johnson. Zimmer, Inc., Warsaw, IN.
- ↑ Ng L, Saul T, Lewiss RE. Sonographic baseline physeal plate width measurements in healthy, uninjured children. Pediatr Emerg Care. 2014 Dec;30(12):871-4. doi: 10.1097/PEC.0000000000000290. PMID: 25407037.
- ↑ Edmond T, Laps A, Case AL, O'Hara N, Abzug JM. Normal Ranges of Upper Extremity Length, Circumference, and Rate of Growth in the Pediatric Population. Hand (N Y). 2020 Sep;15(5):713-721. doi: 10.1177/1558944718824706. Epub 2019 Feb 1. PMID: 30709325; PMCID: PMC7543216.
- ↑ Öztürk A, Çiçek B, Mazıcıoğlu MM, Zararsız G, Kurtoğlu S. Wrist Circumference and Frame Size Percentiles in 6-17-Year-Old Turkish Children and Adolescents in Kayseri. J Clin Res Pediatr Endocrinol. 2017 Dec 15;9(4):329-336. doi: 10.4274/jcrpe.4265. Epub 2017 May 17. PMID: 28515034; PMCID: PMC5785639.
- ↑ Werz SM, Zeichner SJ, Berg BI, Zeilhofer HF, Thieringer F. 3D printed surgical simulation models as educational tool by maxillofacial surgeons. Eur J Dent Educ. 2018;22(3):e500–5. https://doi.org/10.1111/eje.12332.
- ↑ Legocki AT, Duffy-Peter A, Scott AR. Benefits and Limitations of Entry-Level 3-Dimensional Printing of Maxillofacial Skeletal Models. JAMA Otolaryngol Head Neck Surg. 2017;143(4):389–394. doi:10.1001/jamaoto.2016.3673
- ↑ Liu, K., Madbouly, S. A., Schrader, J. A., Kessler, M. R., Grewell, D. and Graves, W. R. (2015), Biorenewable polymer composites from tall oil-based polyamide and lignincellulose fiber. J. Appl. Polym. Sci., 132, 42592. doi: 10.1002/app.42592.
- ↑ Mills, C. A.; Navarro, M.; Engel, E.; Martinez, E.; Ginebra, M. P.; Planell, J.; Errachid, A.; Samitier, J. J. Biomed. Mater. Res. A 2006, 76A, 781.
- ↑ Auras, R.; Harte, B.; Selke, S. Macromol. Biosci. 2004, 4, 835.
- ↑ Snelling PJ, Keijzers G, Ware RS. Point-of-Care Ultrasound Pronator Quadratus Hematoma Sign for Detection of Clinically Non-Angulated Pediatric Distal Forearm Fractures: A Prospective Cohort Study. J Ultrasound Med. 2022 Jan;41(1):193-205. doi: 10.1002/jum.15695. Epub 2021 Mar 10. PMID: 33749859.