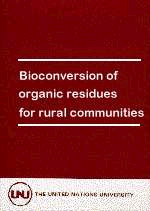
Bioconversion of Organic Residues for Rural Communities (UNU, 1979, 178 p.)
Possible applications of enzyme technology in rural areas[edit | edit source]
Charles L. Cooney
Department of Nutrition and Food Science, Massachusetts Institute of Technology, Cambridge, Massachusetts, USA
Introduction[edit | edit source]
Enzyme technology, in its broadest sense, involves the problems not only of application of the enzymes themselves, but also their production, isolation, and frequently their modification to achieve stability All of these technology components must be considered in the application of enzyme technology. The rationale for development of enzyme technology is based upon the unique advantages of enzymes as catalysts. These advantages include the highly stereo-specific reactions that are catalyzed with few, if any, side reactions, and the rapid reaction rates that are achieved under mild conditions of pH and temperature. These properties make enzymes particularly desirable for processing natural products for eventual human or animal consumption. For this reason, enzyme technology has had substantial impact on the food industry.
However, enzyme catalysis is not without its disadvantages. Enzymes themselves are sensitive to extremes in pH or temperature. In many applications, enzymes are used only once and then remain, usually denatured, with the final product. As a consequence, the cost of enzyme isolation can be quite high. Lastly, when using enzymes over long periods of time, stability becomes of critical importance If subjected to unfavourable conditions, enzymes rapidly lose their catalytic ability.
Enzyme technology has quickly become a very "high technology," or technologically intensive. Herein lies the major limitation to the application of enzyme technology in rural areas. How is it possible to deliver and routinely use enzymes under variable conditions? The major question becomes: "What have we learned about enzyme technology and the properties of enzymes that can now be directed towards the development of simple technological processes that will better the life of small groups of people in simple, rural environments?" This is the challenge Can we use high technology to develop low-technology processes?
Biocatalytic processes[edit | edit source]
Biocatalytic processes may be defined quite broadly, as illustrated in Table 1. Such processes include fermentation, enzyme catalysis, and whole cell systems It is interesting to reflect on the historical flow of this technology. Clearly, the earliest form of biocatalytic processes are represented by fermentation itself, both aerobic and anaerobic. In using such processes, the fermentation industry has developed, and continues to create and expand, new technologies related to pharmaceuticals, foods, and chemicals. However, as our understanding of microorganisms, and enzymes in particular, increased, it became possible to develop enzyme catalysis as a process technology in itself. This has taken place in the form of homogeneous catalysis using freely soluble enzymes for a wide range of applications in the food and pharmaceutical industries (1,2).
TABLE 1. Biocatalytic Processes
Fermentation | aerobic |
anaerobic | |
Enzyme catalysis | homogeneous |
heterogeneous | |
Whole cell systems | cell suspensions |
packed ceils | |
immobilized cells |
Primarily as a consequence of the high cost of enzyme isolation and the sensitivity of enzymes to denaturation, technology has been developed for the immobilization of enzymes onto water-insoluble supports (3). This technology has opened the whole field of heterogeneous catalysis to enzyme catalysts. The use of immobilized enzymes has greatly minimized the cost of production by permitting repeated use of the enzymes, and has substantially increased the stability of the enzymes themselves. This technology has had major impact on the food and pharmaceutical industries and will clearly expand its importance in the years to come.
However, an interesting trend is occurring in the development of biocatalytic processes and that is the use of whole cell systems (4). The cells may be in the form of simple cell suspensions, or they may be packed in beds. Building on the development of immobilized enzymes, a technology has been developed to immobilize whole cells to achieve both stability and process control. In a sense, our development of sophisticated technology and our understanding of enzyme processes has allowed us to go full circle. The use of whole cell systems obviates enzyme isolation and immobilization, but presents the challenge of how to develop microbial cell systems with highly focused reactions and, returning to the original question: How can such systems be used to better life in rural areas?
The major class of enzyme reactions offering the most immediate possibilities for application to bioconversion in rural areas are the hydrolytic enzymes. The use of hydrolytic processes makes thermodynamic sense; such processes take advantage of the fact that hydrolysis of natural polymers in a predominantly aqueous environment has a free energy flow in the direction of hydrolysis. Such processes are usually carried out by one or a few enzymes, they do not require biological co-factors, and they permit de-polymerization under very mild conditions, thereby minimizing side-product formation. Furthermore, the substrates are readily available in rural communities. These substrates include cellulose, starch, hemicellulose, proteins, and many heteropolysaccharides that are present in plant materials.
The utilization of hydrolytic processes can be of benefit by improving current processes that are in use in rural communities; an example is the removal of mucilage from the coffee bean prior to drying (5). Or, it may be possible to use dextranases to eliminate the dextrans that form during prolonged harvesting times or improper storage of sugar-cane before sugar extraction. In a broader sense, it may be possible to use such hydrolytic processes to expand the available raw material base used in food or feed production. For instance, the hydrolysis of starch in products such as cassava, and the hydrolysis of cellulosic materials are possible routes for the production of sugar syrups for immediate use or for further processing.
In the remainder of this paper, I would like to focus on two applications of enzyme technology that might be suitable in rural areas but, more importantly, these two applications illustrate a broad class of possible use. In the first application, emphasis is on the use of enzymes in starch hydrolysis; in the second, it is on the use of whole cell systems in cellulose hydrolysis.
Enzyme hydrolysis of manioc[edit | edit source]
The manioc plant has become of increasing interest as a consequence of its high starch content in tuberous roots that can be propagated in a wide variety of environments (6). Of the two varieties of manioc, the bitter and the sweet, the bitter varieties are of particular interest for cultivation for industrial use because of their higher starch content The starch content of these roots varies according to soil and climatic conditions, but is generally in the range of 20 - 40 per cent; the remaining 60 - 75 per cent is moisture with about 5 per cent of protein, cellulose, minerals, etc. (7). Manioc can provide a low-cost source of starch for food uses and is important for this reason, and it is of increasing importance to industry for the same reason. In many applications, it is desirable first to hydrolyze the starch to produce soluble sugars for subsequent use.
To this end, Lages and Tannenbaum have recently developed a process for using an extremely thermostable a-amylase for liquefaction of cassava starch followed by a high temperature treatment with amylogiocosidase (8). While starch hydrolysis is itself not new, the utilization of high temperature for hydrolysis has several advantages. These include rapid liquefaction of the starch and pasteurization of the product because of the elevated temperature.
In addition, it has been shown by Lages and Tannenbaum (8) that, following the thermoamylase treatment, the requirements for amyloglucosidase are greatly reduced, as is the time for complete conversion of the starch to sugar. The a-amylase employed was produced from Bacillus licheniformis (Thermamyl Liquid 60; Novo Industrials, Bagsvaerd, Denmark) added to either crude tapioca or cassava meal. Starting solutions of the starches were prepared by adding 33 g of material to enough water to give a total weight of 100 9. After preparation of these slurries, the a-amylase was added and the mixture was heated to 105°C for six minutes, after which the temperature was reduced to 95 C for two hours. At the completion of liquefaction, the temperature was reduced to 60 C and amyloglucosidase (AMG 150, liquid; Novo Industrials) was added.
The kinetics and conversion in such a process, in which the authors used an optimal amount of amyloglucosidase (0.5 per cent V/W) to achieve 100 per cent conversion in less than 24 hours, are illustrated in Figure 1. The resulting product from this hydrolysis can be used directly as a sugar syrup, or it can provide the substrate for subsequent fermentations to desired products, including single-cell protein and alcohol for fuel use (9).
Figure. 1. Kinetics of Tapioca Starch Hydrolysis with Amylo-Glucosidase (0.025 and 0.05 per cent V/W) (From Lages and Tannenbaum [8])
The desirability of carrying out the starch hydrolysis before the production of single-cell protein is based upon the rationale that one can now use widely acceptable food yeasts, e.g., Saccharomyces cerevisiae or Candida utilis, rather than having to develop microbial species with amylolytic activity. These sugar syrups would also be quite suitable for making such products as baker's yeast. However, this approach to enzyme application implies the availability of the enzyme, which may, in fact, be the major limitation in the application of enzyme technology in the rural community.
Whole cell systems[edit | edit source]
An alternative to the use of purified enzymes is the application of whole cell systems that can be achieved by a variety of means (4). The whole cell represents a "packaged enzyme" or "enzyme system." Most important, by means of the whole cell, it is possible to use sophisticated technology to develop desired cell lines, e.g., through techniques such as genetic engineering, that will carry out highly specific processes under specific conditions. The ability of the cells to grow and reproduce permits the rapid transfer of this technology into the field. The end" user is not concerned with how whole cell systems were developed, but with how they can be used. Desired cell lines carrying out specific functions can be distributed by means of marketing starter cultures, or the technology of cell propagation can be achieved at the local level. An example of one aspect of this approach is the fermentation of cellulose to produce utilizable sugars in a single-step operation.
Cellulose degradation and utilization[edit | edit source]
In examining the prospects for a simple process for the degradation and utilization of cellulose, we chose to look at the thermophilic anaerobic bacterium, Clostridium thermocellum. This organism grows well at temperatures of 60 C and above. Most important, it has been observed that this organism has the unique capability to accumulate sugars while degrading and growing on cellulose (10). This phenomenon is illustrated in Figure 2. In this example, medium containing 11 g per litre of solka-floc was inoculated with C. thermocellum. During the course of the fermentation, there is a rapid accumulation of reducing sugars approximately in parallel with growth and cellulose (expressed as CMCase) production From the degradation of approximately 10 g/l of cellulose over the course of 60 hours, there is an accumulation of almost 7 g/l of reducing sugars. Thus, one achieves approximately a 65 per cent yield of reducing sugars from the cellulose that is hydrolyzed
Figure. 2. Fermentation of Cellulose with Accumulation of Reducing Sugar by Clostridium thermocellum at 60 C (From Cooney et al. [13])
When the sugar products are examined by means of high pressure liquid chromatography (HPLC) (Figure 3), the predominant products are glucose and cellobiose. When C. thermocellum is grown on natural cellulosic materials containing hem-cellulose, there is also an accumulation of xylose and possibly xylobiose. This observation is particularly interesting, because C. thermocellum will not use the pentoses for growth.
Figure. 3. Accumulation of Glucose and Cellobiose during In Vivo Saccharification of Cellulose by Clostridium thermocellum
We examined both cellulase and xylanase activity in cell-free broths from C. thermocellum grown on a variety of substrates, as shown in Table 2. When C. thermocellum was grown on these substrates, the ratio of xylanase to CMC's activity was consistently one. These results suggest that cellulose activity and xylanase activity are caused by the same enzymes. This observation has been made in other organisms (11), and the dual activity of the cellulase enzymes would account for the simultaneous accumulation of pentoses and hexoses.
TABLE 2. Production of Xylanase and Cellulose Activity by C. thermocellum Grown on Selected Carbon Sources
Xylanase* mg/ml/hr |
CMCase* mg/ml/hr |
Xylanase CMCase | |
Substrate | |||
Sorka-floc | 7.0 | 6.75 | 1.04 |
Corn stover | 1.95 | 1.9 | 1.03 |
Avicel | 3.0 | 3.2 | 0.94 |
Cotton | 4.8 | 5.0 | 0.96 |
* The variation in the values among the substrates reflects the time when the fermentations were ended, as well as C. thermocellum's ability to grow and produce enzymes on the particular substrate.
In an attempt to improve and optimize the process, we examined the influence of pH control during the course of the fermentation. In the previous experiments, pH was set initially at 6.8 and then left to fall during the course of the fermentation. Results shown in Table 3 illustrate the difference in performance for C.thermocellum with controlled pH (6.8) and uncontrolled pH fermentations on cellulose. As seen by the results in this table, with pH control there is greater degradation of cellulose, increased cell mass formation, increased synthesis of fermentation products, e.g., ethanol and acetic acid, but markedly less accumulation of reducing sugar. These results suggest that the marked sugar accumulation during growth on cellulose results at least in part from a restriction in growth, probably by the decreased pH, in the presence of excess cellulase capacity. Hence, cellulose is hydrolyzed at a rate faster than it can be utilized.
TABLE 3. Comparison of Results from pH-Controlled and Non-pH-Controlled Fermentations of C thermocellum Grown on Solka-Floc
Non-pH -controlled | pH-controlled | |||
Initial | Final | Initial | Final | |
pH | 6.8 | 5.7 | 6.8 | 6.8 |
Cellulose, g/l | 10.1 | 2 4 | 10.0 | 1.8 |
Dry cell weight, g/l | - | 0.5 | - | 0.8 |
Reducing sugar, g/l | 0.3 | 6.0 | 0.1 | 2.4 |
Ethanol, g/l | - | 0.4 | - | 1.3 |
Acetic acid, g/l | - | 1.0 | - | 2.7 |
The approach illustrated by the above results offers the opportunity for in vivo saccharification of ligno-cellulosic materials to accumulate sugars, as well as the possibility for a direct fermentation for product formation from cellulose. For example, C. thermocellum will produce ethanol, acetic acid, and lactic acid directly from cellulose. Product accumulation is shown in Figure 4, which presents results from C. thermocellum grown on cellulose added intermittently during the course of fermentation. This allowed an increased amount of cellulose to be acided to the broth. A total of 20 9 of cellulose were added, and this resulted in the production of 8.5 g/l of reducing sugars and 4 g/l each of ethanol and acetic acid.
Figure. 4. Kinetics of Cellulose Hydrolysis and Ethanol and Acetic Acid Production in a Fedbatch Fermenter with Clostridium thermocellum (From Cooney et al. 113])
The above example illustrates the direct conversion of cellulose to ethanol. Through genetic manipulation, it is possible to minimize the amount of acetic acid, which is usually produced in molar ratio of 1:1 with ethanol, so that the ethanol-acetic acid ratio becomes 8:1 (12). This is an example of what one can do with high technology to manipulate the ceil in order to generate a cell line with some desired properties, in this case, over-production of ethanol directly from cellulose. One of the bottlenecks in this fermentation is the sensitivity of C. thermocellum to ethanol and aceitic acid. A eel) line that is tolerant to ethanol concentrations of approximately 5V per cent has been developed, and thus we have again modified this cell line to achieve a desired final objective (12).
Transfer of enzyme technology to rural communities[edit | edit source]
The above examples illustrate the possibilities of using enzyme technology to achieve new or improved use of natural products such as starch and cellulose. In the example of starch hydrolysis, it is possible to produce a fermentable syrup that can be used to cultivate acceptable food and feed yeasts. The major limitation, however, is the lack of the required enzymes in the rural community.
In the second example, a fermentation directly on cellulase that will allow both accumulation of enzymes via saccharification and production of useful products is described. This approach is particularly exciting because it offers a method by which enzyme unavailability can be overcome. The micro-organisms that contain the desired sets of enzyme activity readily reproduce themselves. Therefore, it is possible to develop, via high technology in the laboratory, strains of micro-organisms that have the desired properties and then take these organisms into the field where they can be reproduced repeatedly and used on a local basis. In this manner, the microbial cell becomes the vehicle for transferring high technology to the community.
Examining further the example of cellulose hydrolysis, it is possible to develop a system for saccharification of cellulose for sugar production, or a system for the direct conversion of cellulose to ethanol, acetic acid, or lactic acid. Alternatively, it is possible to add a second microbial culture that would utilize the sugars released by C. thermocellum-mediated cellulose saccharification. The resulting mixed culture could be used to produce lactic acid for use as a food preservative, short-chain fatty acids for animal feeding, or solvents for use in extraction of oilseed meals. Furthermore, via genetic engineering, it should be possible to incorporate traits in these organisms to make them withstand the highly competitive environment encountered in the rural community. The increased ability to compete with indigenous organisms is important so that fermentations can be run with little or no sterilization, under conditions of variable pH and temperature, or in combination with other desired organisms.
The key question raised earlier, of how to transfer and propagate enzyme technology in the field, could be answered by using starter cultures either prepared at a central location or propagated by a serial transfer using techniques similar to those used for traditional food fermentations. An interesting alternative would be to take advantage of the concept of immobilized whole cells. For this purpose, one could use readily available inorganic supports, such as porous rocks. One may choose to use high carbonate rocks to exert some pH control of the fermentation, or simply volcanic rock with a high degree of porosity. The microorganisms present in the system would adsorb to, and become associated with, the solid phase so that after removal of the fermentation liquid the organisms would be left behind. This would serve to help build up a substantial active population of microorganisms, as well as serially transfer the desired population from fermentation to fermentation, even from family to family and village to village.
Conclusions[edit | edit source]
In conclusion, it is evident from this examination of the possible applications of enzyme technology to rural communities, that any system must employ very simple technology, and that high-technology whole cell systems could be used to develop desired cell lines, while the micro-organisms themselves could provide the mechanism for transfer of this technology to the field. Thus, the application of technologies such as genetic engineering, mixed culture development, and microbial selection could be used to create desired micro-organisms that would then be self-replicating units within the rural community. it is likely that hydrolytic processes would be the first to have immediate impact in world development. This is based on the availability of a wide variety of natural polymers whose hydrolytic degradation would either improve existing technologies or expand the raw material availability to the rural community.
Lastly, the development of starter culture processes for propagation of these cell lines, and/or the implementation of in situ cell recycling by natural immobilization of cells onto porous inorganic materials, offer a possibility for improving and propagating this technology. The use of whole cell systems provides much potential for the application of fermentation and enzyme technology to the improvement of life in the rural community.
References[edit | edit source]
1. R.A. Messing, Immobilized Enzymes for Industrial Reactors Academic Press, New York, 1975.
2. A.C. Olson and C.L. Cooney, Immobilized Enzymes in Food and Microbial Processes, Plenum Press, New York,1974,
3. O.R. Zaborskv, Immobilized Enzymes, CRC Press, Cleveland, Ohio,1973.
4. T.R. Jack and J.E. Zajic, "The immobilization of Whole Cells," Adv. Biochem. Eng. 5: 125 (1977).
5. J.F. Menchu and C. Rolz, "Coffee Fermentation Technology," paper presented at the American Chemical Society Meeting in Washington, D.C., 13 - 17 Sept. 1971, (unpublished),
6. AID Contract No. csd/2497. A Literature Review and Research Recommendations on Cassava, 1972.
7. J.C. Ayres, "Manioc: The Potential Exists for Increased Use of This Tropical Plant and Its Products," food Technol. 26: 128 (1972).
8. A.C.A. Lages and S.R. Tannenbaum, "Production of Glucose from Tapioca (Cassava Starch) and Farinha de Mandioca (Cassava Meal)," J. food Sci. 43: 1012 (1978)
9. G.G. Teixeira, "Produsao de alcool de Mandioca," Bragantia 10:278 (1950).
10. J. Gordon, M. Jiminez, C.L. Cooney, and D.I.C. Wang, "Sugar Accumulation during Enzyme Hydorlysis and Fermentation of Cellulose, "AIChE Symposium Series No.181, 74: 91 (1978).
11. I.V. Gorbacheva and N.A. Rodionova, "Studies on Xylan Degrading Enzymes. I. Purification and Characterization of endo-1 -4-ß-Xylanase from Aspergillus niger str. 14, " Biochem. Biophys. Acta 484: 79 (1977).
12. S-D. Wang, "Production of Ethanol from Cellulose by Clostrium thermocellum," Master of Science Thesis, Department of Nutrition and Food Science, Massachusetts Institute of Technology, Cambridge, Massachusetts, 1979.
13. C.L. Cooney, D.I .C. Wang, S -D. Wang, J. Gordon, and M. Jiminez, "Simultaneous Cellulose Hydrolysis and Ethanol Production by a Cellulolytic Anaerobic Bacterium," Biotech. Bioeng. Symposium Series No.8. (in press.)
Discussion summary[edit | edit source]
It was pointed out that the objective of using mixed cultures to degrade cellulose is to accelerate the production of other products such as lactic acid or butanol, not to increase sugar yields.
The question was raised as to whether modifications could be introduced that would make more robust strains of cellulolytic organisms better suited to fermentation processes in rural communities. Although this may be possible, much more research needs to be done.
Professor Cooney was asked whether he had used a mixed culture, taking advantage of sugar-accumulating properties of Clostridium thermocellum combined with a methane-producing bacterium. This kind of methane production has been pursued successfully in Wisconsin, but the MIT work with mixed cultures has concentrated on production of more valuable products. It was noted that there are many ways in which advanced research in industrialized countries can produce results adaptable for use in rural communities.